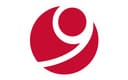
MED-EL
Published Jun 25, 2024
9 Reasons Why Cochlear Implant Electrodes Matter
A cochlear implant electrode serves not only as a crucial bridge between technology and nature that connects recipients to sound. The electrode array can significantly impact hearing outcomes. For instance, there is now growing consensus among researchers that stimulating more of the cochlea is associated with better speech perception and music appreciation. Here is the latest research you need to know to ensure each of your patients can benefit the most from their cochlear implant.
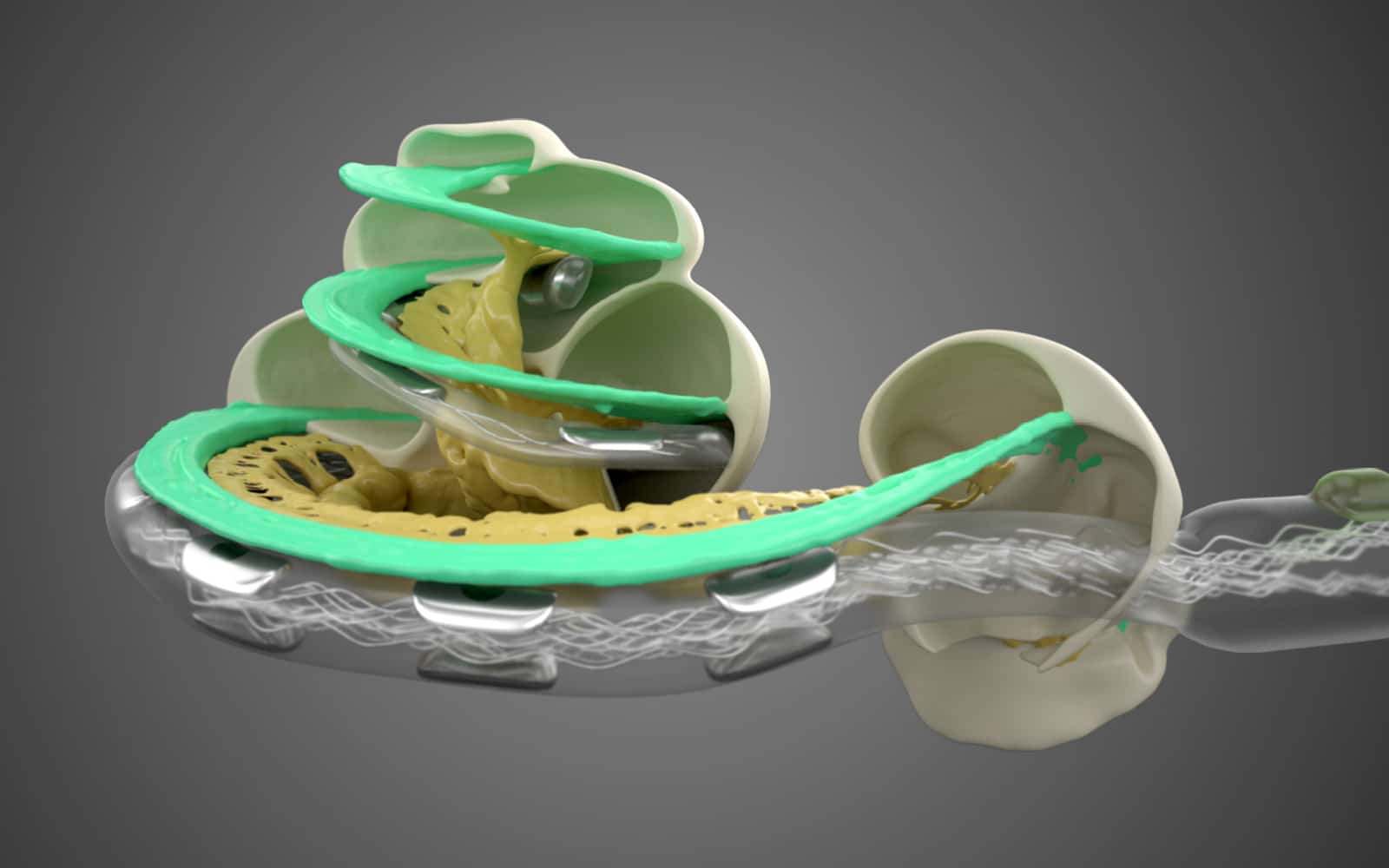
- 1 Hearing Structures Extend Beyond 1.5 Turns
- 2 Better Speech Perception
- 3 More Natural Sound Quality
- 4 Musical Enjoyment
- 5 Cochleae Naturally Vary in Size and Shape
- 6 Even the Smallest Cochlea Can Accommodate a FLEX Electrode
- 7 Every Patient Can Receive an Electrode to Fit Their Cochlea
- 8 Anatomy-Based Fitting May Optimize Outcomes
- 9 Preserve Future Cochlear Health
In 1996, MED-EL launched the COMBI40+ cochlear implant system featuring a 31-mm-long flexible electrode for coverage of the entire length of the cochlea. Since then, MED-EL’s philosophy has always been to adapt our implants to your patients instead of expecting your patients to adapt to their implant.
Today, scientific knowledge of the variation in cochleae shapes and sizes has evolved along with MED-EL’s electrode portfolio and surgical planning software, allowing surgeons and their teams to visualize the ideal electrode to cover each patient’s entire cochlea and make use of its full potential as nature intended. Here you will find nine key ways electrodes affect outcomes according to recent research, along with how surgeons and clinicians can use these insights to optimize their patients’ outcomes with cochlear implants.
1. Hearing Structures Extend Beyond 1.5 Turns in the Cochlea
Studies using advanced high-resolution 3D synchrotron imaging have shown that anatomical structures important for cochlear stimulation, such as the Rosenthal’s canal and spiral ganglion (SG), extend deeper into the cochlea than some experts previously thought.Li, H., Schart-Morén, N., Rohani, S. A., Ladak, H. M., Rask-Andersen, H., & Agrawal, S. (2020). Synchrotron radiation-based reconstruction of the human spiral ganglion: Implications for cochlear implantation. Ear & Hearing, 41(1), 173–181. https://doi.org/10.1097/aud.0000000000000738[1]Li, H., Helpard, L., Ekeroot, J., Rohani, S. A., Zhu, N., Rask-Andersen, H., Ladak, H. M., & Agrawal, S. (2021). Three-dimensional tonotopic mapping of the human cochlea based on synchrotron radiation phase-contrast imaging. Scientific Reports, 11(1). https://doi.org/10.1038/s41598-021-83225-w [2]Helpard, L., Li, H., Rohani, S. A., Zhu, N., Rask-Andersen, H., Agrawal, S., & Ladak, H. M. (2021). An approach for individualized cochlear frequency mapping determined from 3D synchrotron radiation phase-contrast imaging. IEEE Transactions on Biomedical Engineering, 68(12), 3602–3611. https://doi.org/10.1109/tbme.2021.3080116[3]
Synchrotron imaging offers some key advantages when used to view the delicate, miniscule anatomical structures of the human cochlea. First, it allows researchers to bypass the need to undergo staining, which often causes shrinkage. Second, it provides extremely high-resolution images down to the micrometer with high contrast, allowing us to view smaller structures with much more detail and contrast than previously possible.
“The mean SG angular length of cochleae in the sample set was 2.00 turns, or 720 degrees. The minimum and maximum SG angular lengths observed were 1.84 and 2.22 turns, respectively.”
Helpard et al., 2021
Thanks to synchrotron imaging, we now have a more complete understanding of the complexities of cochlear anatomy. One key finding is that the human spiral ganglion extends nearly the full natural angular length of the cochlea. These studies have determined that the average length of the spiral ganglion extends more than one and half turns, and in some cases even more than two turns.
“From an anatomical standpoint, optimal reach of the spiral ganglion would seem to be at two turns, thereby covering the entire frequency range.”
Li et al., 2021
Synchrotron imaging studies have revealed that the spiral ganglion extends deeper than indicated in older studies using other methods. A meta-analysis of publications between 1968 and 2018 that examined the depth of spiral ganglion cell bodies and provided the number of spiral ganglion cell bodies in the four segments of the human cochlea and angular depth distribution of them, found that spiral ganglion cell bodies extend 630-680 degrees, which is also deeper than one and a half turns in the cochlea.Dhanasingh, A. E., Rajan, G., & van de Heyning, P. (2020). Presence of the spiral ganglion cell bodies beyond the basal turn of the human cochlea. Cochlear Implants International, 21(3), 145–152. https://doi.org/10.1080/14670100.2019.1694226[4]
“Spiral ganglion cell bodies inside the Rosenthal’s canal (RC) in the modiolus trunk extended to an angular depth of 630–680° which is near the end of the second turn of the cochlea.”
Dhanasingh et al., 2020
2. Longer Electrode Arrays Associated With Better Speech Perception
A systematic literature review and meta-analysis of studies examining angular insertion depth of cochlear implant electrodes was published in 2023.Breitsprecher, T. M., Baumgartner, W. D., Brown, K., Dazert, S., Doyle, U., Dhanasingh, A., ... & Weiss, N. M. (2023). Effect of Cochlear Implant Electrode Insertion Depth on Speech Perception Outcomes: A Systematic Review. Otology & Neurotology Open, 3(4), e045. https://doi.org/10.1097/ONO.0000000000000045[5] It found that the majority of studies concluded that longer electrode arrays tend to deliver better speech perception. 23 studies met the inclusion criteria, and 15 out of these 23 found either a significant positive correlation or a positive effect between insertion depth and speech perception.
“Deeper insertion of a lateral wall array appears to confer speech recognition benefit up to 600°, with a plateau in performance observed thereafter.”
Canfarotta et al., 2021
A more recent study published after the meta-analysis mentioned above also supports these findings, stating that “patients with deeper insertions perform better in speech recognition tasks.”Weller, T., Timm, M. E., Lenarz, T., & Büchner, A. (2023). Cochlear coverage with lateral wall cochlear implant electrode arrays affects post-operative speech recognition. PLOS ONE, 18(7). https://doi.org/10.1371/journal.pone.0287450[6]
In addition, it also found that patients with deeper insertion saw greater benefit from cochlear implants with respect to their preoperative state.
Speech Perception Scores vs. Insertion Angle
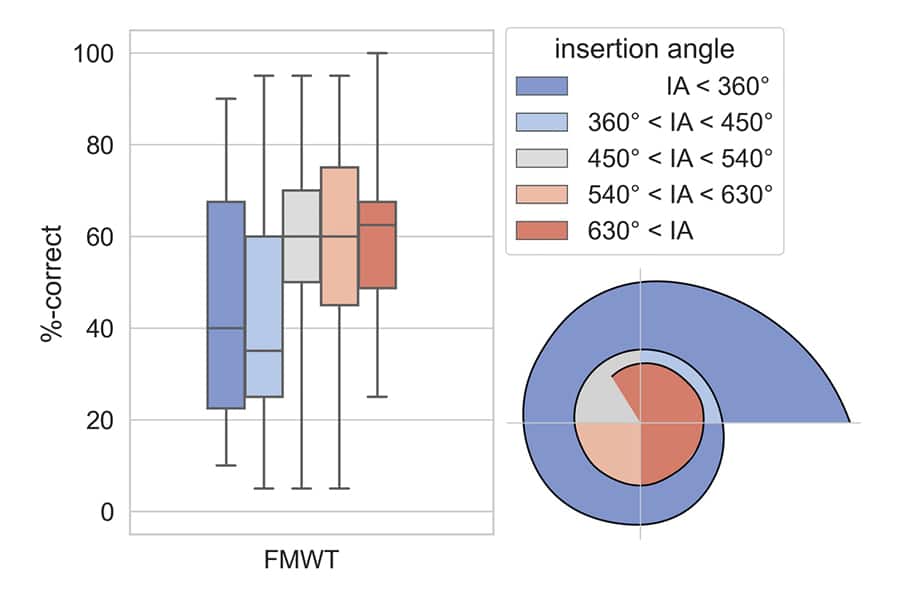
Weller et al., (2023). Speech perception scores on the Freiburger Monosyllabic Word Test (FMWT) were compared between groups of patients based on electrode insertion depth. Here you can see that patients in the group with insertions over 630° had the highest speech perception scores, followed by those with insertions over 540°.
“Benefit is significantly higher for an insertion angle of 540° - 630° compared to below 360°, with no significant decrease after 630°.”
Weller et al., 2023
Thus, when examining speech perception and angular insertion depth, results tend to be better when cochlear implant electrodes stimulate beyond one and a half turns in the cochlea.
3. Deeper Insertion Depth Supports More Natural Sound Quality
Since the natural hearing nerve structures go beyond one and a half turns in the cochlea, it also makes sense that electrodes should cover one and a half to two turns to support restored tonotopic pitch perception. This is because the brain understands the frequency of a sound based on which hair cells at a specific place in the cochlea are stimulated by a sound.Li, H., Schart-Moren, N., Rohani, S., A., Ladak, H., M., Rask-Andersen, A., & Agrawal, S. (2020). Synchrotron Radiation-Based Reconstruction of the Human Spiral Ganglion: Implications for Cochlear Implantation. Ear Hear. 41(1). https://doi.org/10.1097/AUD.0000000000000738[7]
“It is generally believed that frequency-place matching of characteristic frequencies in cochlear implant electrodes to code appropriate neural elements is beneficial for functional outcome.”
Li et al., 2020
If an electrode doesn’t cover the part of the cochlea beyond one and half turns, it can’t accurately stimulate sound frequencies in that area. Thus, stimulating beyond one and half turns is also the key to more natural sound quality with a cochlear implant. In fact, extensive research has shown that this accurate place-pitch matching is the only reliable way to provide more accurate pitch perception with a cochlear implant.Landsberger, D. M., Svrakic, M., Roland, J. T., & Svirsky, M. (2015). The relationship between insertion angles, default frequency allocations, and spiral ganglion place pitch in cochlear implants. Ear & Hearing, 36(5). https://doi.org/10.1097/aud.0000000000000163 [8]Landsberger, D. M., Vermeire, K., Claes, A., Van Rompaey, V., & Van de Heyning, P. (2016). Qualities of single electrode stimulation as a function of rate and place of stimulation with a cochlear implant. Ear & Hearing, 37(3). https://doi.org/10.1097/aud.0000000000000250[9]Li, H., Schart-Morén, N., Rohani, S. A., Ladak, H. M., Rask-Andersen, H., & Agrawal, S. (2019). Synchrotron radiation-based reconstruction of the human spiral ganglion: Implications for cochlear implantation. Ear & Hearing, 41(1), 173–181. https://doi.org/10.1097/aud.0000000000000738[10]McDermott, H., Sucher, C., & Simpson, A. (2009). Electro-Acoustic Stimulation: Acoustic and Electric Pitch Comparisons. Audiology and Neurotology, 14(Suppl. 1), 2–7. https://doi.org/10.1159/000206489[11]Roy, A. T., Penninger, R. T., Pearl, M. S., Wuerfel, W., Jiradejvong, P., Carver, C., Buechner, A., & Limb, C. J. (2016). Deeper cochlear implant electrode insertion angle improves detection of musical sound quality deterioration related to bass frequency removal. Otology & Neurotology, 37(2), 146–151. https://doi.org/10.1097/mao.0000000000000932[12]Schatzer, R., Vermeire, K., Visser, D., Krenmayr, A., Kals, M., Voormolen, M., Van de Heyning, P., & Zierhofer, C. (2014). Electric-acoustic pitch comparisons in single-sided-deaf cochlear implant users: Frequency-place functions and rate pitch. Hearing Research, 309, 26–35. https://doi.org/10.1016/j.heares.2013.11.003[13]Rader, T., Döge, J., Adel, Y., Weissgerber, T., & Baumann, U. (2016). Place dependent stimulation rates improve pitch perception in cochlear implantees with single-sided deafness. Hearing Research, 339, 94–103. https://doi.org/10.1016/j.heares.2016.06.013[14]
Natural Pitch Matching With MED-EL FineHearing
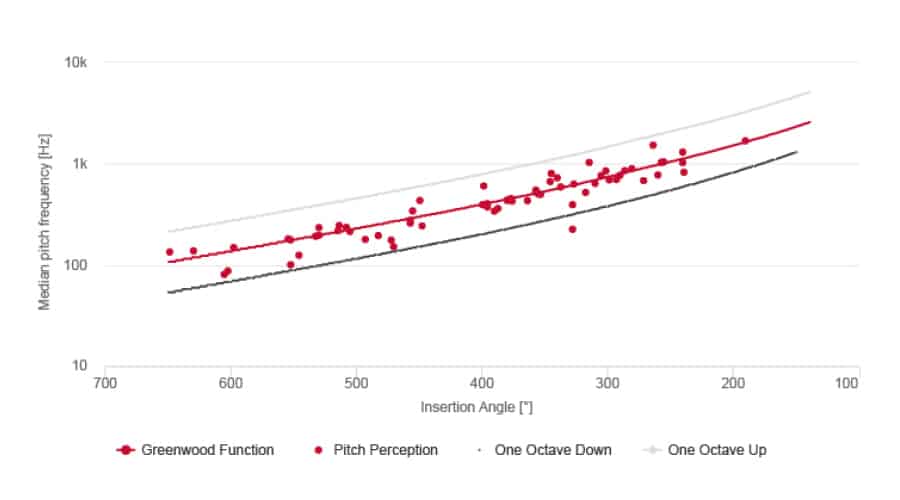
MED-EL cochlear implant sound coding mimics the natural time-coding for low frequencies and provides place-pitch match throughout the cochlea. By mimicking natural sound coding, FineHearing provides much more natural sound quality.
Place dependent stimulation rates “demonstrate thus far unparalleled restoration of tonotopic pitch perception in CI users.”
Rader et al., 2016
In addition to accurate place-pitch matching, rate-based coding,Schatzer, R., Vermeire, K., Visser, D., Krenmayr, A., Kals, M., Voormolen, M., Van de Heyning, P., & Zierhofer, C. (2014) Electric-acoustic pitch comparisons in single-sided-deaf cochlear implant users: frequency-place functions and rate pitch. Hear Res., 309, 26–35. https://doi.org/10.1016/j.heares.2013.11.003[35] FineHearing sound coding strategies, and “phase locking” in the apical region also play important roles in supporting more natural sound quality.
4. Deeper Electrode Insertion Supports Musical Enjoyment
When electrode arrays reach beyond one and a half turns of the cochlea, they can stimulate the crucial low frequency portions of the cochlea. As we will show later, MED-EL electrode arrays are the only electrodes that can reach beyond one and a half turns in the cochlea to stimulate the low frequencies at their natural tonotopic place in the cochlea. This increased apical cochlear stimulation allows MED-EL cochlear implant users to hear the deeper bass tones.Roy, A. T., Carver, C., Jiradejvong, P., & Limb, C. J. (2015). Musical Sound Quality in cochlear implant users. Ear & Hearing, 36(5), 582–590. https://doi.org/10.1097/aud.0000000000000170[15]Roy, A. T., Penninger, R. T., Pearl, M. S., Wuerfel, W., Jiradejvong, P., Carver, C., Buechner, A., & Limb, C. J. (2016). Deeper cochlear implant electrode insertion angle improves detection of musical sound quality deterioration related to bass frequency removal. Otology & Neurotology, 37(2), 146–151. https://doi.org/10.1097/mao.0000000000000932[16]
“Cochlear implant users with greater apical stimulation made sound quality discriminations that more closely resembled those of [normal hearing] controls for stimuli that contained low frequencies.”
Roy et al., 2016
Thanks to our advanced sound coding strategies and long electrodes, our cochlear implants help users to hear every tone and the fine details of their favorite songs. This makes MED-EL cochlear implants the ideal cochlear implant for music.
“After losing my hearing, it was difficult to listen to music. And now with the cochlear implant, I can hear more overtones and undertones. I appreciate music to the fullest.”
Russell Tyler
MED-EL cochlear implant recipient and oboist
5. Cochleae Naturally Vary in Size and Shape
Another recent meta-analysis and systematic literature review recently quantified the variation of eight cochlear dimensions from 33 studies that met the inclusion criteria.Curtis, D. P., Baumann, A. N., & Jeyakumar, A. (2023). Variation in cochlear size: A systematic review. International Journal of Pediatric Otorhinolaryngology, 171, 111659. https://doi.org/10.1016/j.ijporl.2023.111659[17] With data from 5,791 cochleae, the study summarized the weighted mean and range values, including cochlear duct length and cochlear height. The mean cochlear duct length was calculated to be 33.04 mm, with a range from 28.2–36.4 mm (n = 2252) and in terms of cochlear height, the mean value is 5.14 mm, ranging from 2.8–6.9 mm (n = 2098).
“We know there’s a huge variation in sizes from cochlear lengths. The organ of Corti, the cochlear duct length varies from 25– 36 millimeters. Really this is a 50% change, from one patient to another patient. This is a huge advantage that we can individualize the choice of these electrode lengths.”
Prof. Paul van de Heyning
As mentioned before, our philosophy is straightforward: Adapt the implant and electrode to your patient instead of adapting your patient to the implant. No other cochlear implant manufacturer offers such an extensive variety of electrodes, allowing surgical teams to easily optimize the electrode array based on the measurements of each individual cochlea.
That’s why we encourage surgical teams to plan cochlear implantations with OTOPLAN, our intuitive surgical planning software, to preoperatively visualize the electrode based on the measurements of each patient’s individual cochlea. With six FLEX arrays available in sizes between 20–34 mm, you can achieve cochlear coverage and full electrode insertion beyond one and a half turns for each patient.
FLEX Electrodes: Individualized Cochlear Implants to Fit Each Cochlea
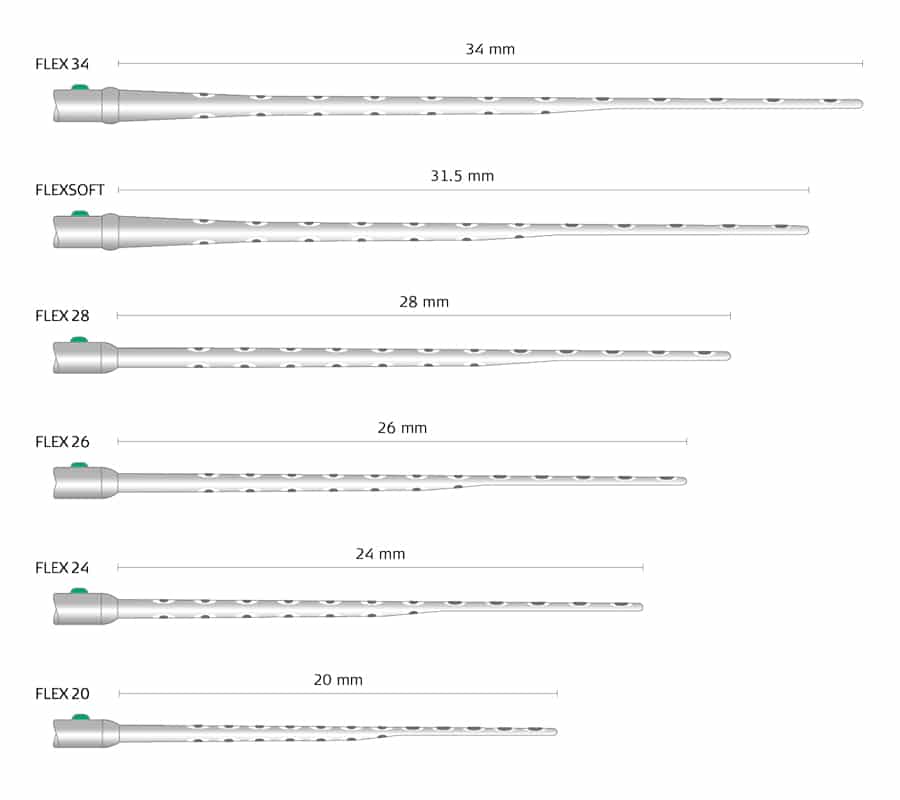
The variability in cochlear anatomy from patient-to-patient results in the same electrode model achieving a different angular insertion depth for each patient. For example, when 47 cochleae implanted with a MED-EL FLEXSOFT electrode were examined, it was found that the mean angular insertion depth was 628 degrees, ranging from 581 to 675 degrees.Canfarotta, M. W., Dillon, M. T., Brown, K. D., Pillsbury, H. C., Dedmon, M. M., & O’Connell, B. P. (2021). Insertion depth and cochlear implant speech recognition outcomes: A Comparative Study of 28- and 31.5-mm lateral wall arrays. Otology & Neurotology, 43(2), 183–189. https://doi.org/10.1097/mao.0000000000003416[18]
“Small cochlea size corresponded to higher insertion angle and reduction of tonotopic mismatch on a 28-mm-long straight lateral wall electrode array. Tonotopic mismatch could be minimized preoperatively by choosing electrode arrays according to the individual cochlear morphology and postoperatively by appropriate frequency fitting.”
Dutrieux et al., 2021
The means and standard deviation of post-operative angular insertion depths of electrodes available on the market are shown below. Results are shown in the graph below, with the bars showing the mean values and the standard deviation indicated by the black whiskers. There you can see that MED-EL FLEX28 and FLEXSOFT electrodes, on average, can achieve insertion depths beyond one and a half turns.
Only MED-EL Electrodes Can Reach Beyond One And a Half Turns
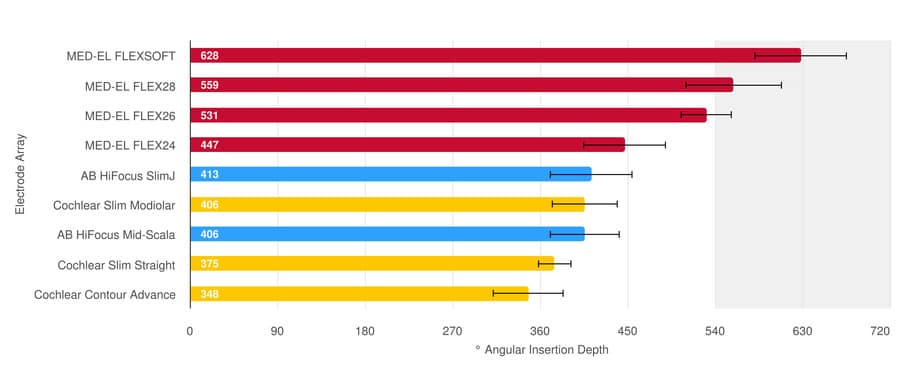
Note: FLEX24 electrodes are typically implanted in patients for which electric-acoustic stimulation is appropriate and a study with our new FLEX34 electrode, which has recently been released on the market, has not yet been published. Source: Hassepass et al, 2014; Downing 2018; Ketterer et al. 2018; McJunkin et al. 2018; Skarzynski et al. 2018; Weller at al. 2023; Canforotta et al. 2021.
"Almost three-fourths of scientific reports evaluating the effect of electrode angular insertion depth on hearing outcomes confirm increased hearing benefits associated with electrical stimulation covering both basal and middle turns of the cochlea. This can be explained by facts like electrodes covering a broader frequency range, stimulating a larger number of neuronal cell bodies that are distributed up to 680° of angular insertion depth, greater spatial separation between adjacent electrode contacts, and closer matching of electrical with place-equivalent acoustic pitches."
Dhanasingh et al., 2024
6. Even the Smallest Cochlea Can Accommodate a FLEX Electrode
MED-EL’s FLEX electrodes feature FLEX-Tip technology. They were all designed with a tapered, rounded tip that gently glides along the scala tympani during insertion, avoiding deviation into the scala vestibuli and damage to the basilar membrane.Dhanasingh, A. & Jolly, C. (2019). Review on cochlear implant electrode array tip fold-over and scalar deviation. J Otol. 14(3). 94-100. https://doi.org/10.1016/j.joto.2019.01.002[19]
In addition, recent synchrotron imaging has also shown that even in the smallest cochleae, the scala tympani can accommodate MED-EL FLEX electrode arrays up to two full turns.Micuda, A., Li, H., Rask‐Andersen, H., Ladak, H. M., & Agrawal, S. K. (2024). Morphologic analysis of the Scala tympani using synchrotron: Implications for cochlear implantation. The Laryngoscope. https://doi.org/10.1002/lary.31263[20]
FLEX Electrodes: The Thinnest Full-Length Electrodes for Cochlear Implants
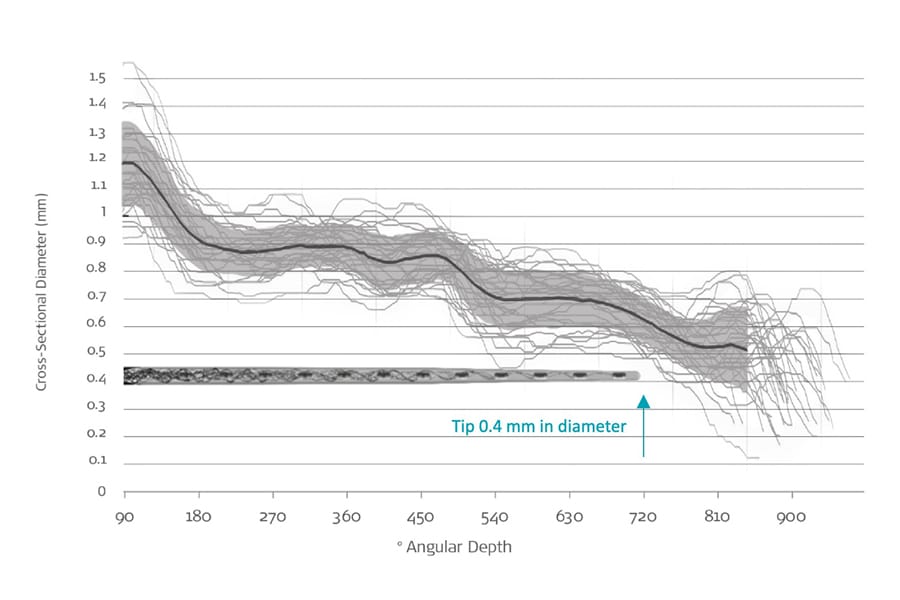
Micuda et al., 2024. Synchrotron Scala Tyampani Measurements
“This study represents a wide variability in cochlear morphology and suggests that even in the smallest cochlea, the scala tympani can accommodate a 0.4 mm diameter electrode up to 720°. Additionally, all lateral wall array trajectories were within the anatomically accommodating insertion zone.”
Micuda et al., 2024
7. Every Patient Should Receive an Electrode That Fits Their Cochlea
To achieve an insertion beyond one and a half turns to find the optimal fit for each patient’s cochlea, OTOPLAN can help surgical teams with preoperative planning and visualization of the optimal electrode. OTOPLAN measures the dimensions of each individual cochlea using preoperative imaging, making a virtual 3D reconstruction of each ear. With this 3D reconstruction, including important parameters such as cochlear duct length and cochlear height, the surgical team can perform virtual electrode insertions to estimate cochlear duct length and select an electrode that is a good fit.Koch, R. W., Ladak, H. M., Elfarnawany, M., & Agrawal, S. K. (2017). Measuring cochlear duct length – a historical analysis of methods and results. Journal of Otolaryngology - Head & Neck Surgery, 46(1). https://doi.org/10.1186/s40463-017-0194-2[21]
“3D reconstruction provides excellent visualization of the cochlea and avoids errors evident in other methods.”
Koch et al., 2017
The 2024 release of OTOPLAN allows you to quicky reconstruct the full inner ear in 3D including the cochlea, scala tympani, scala vestibuli, round window membrane, bony overhang, semicircular canals, and internal auditory canal.
With this 3D reconstruction, your surgical team can perform virtual 3D scala tympani insertion to visualize insertion depth and frequency coverage of each MED-EL electrode. It also allows for planning the optimal placement of the implant housing and audio processor, which is especially helpful for bilateral patients who would like the optimal cosmetic outcome.
8. Anatomy-Based Fitting May Further Optimize Hearing Outcomes
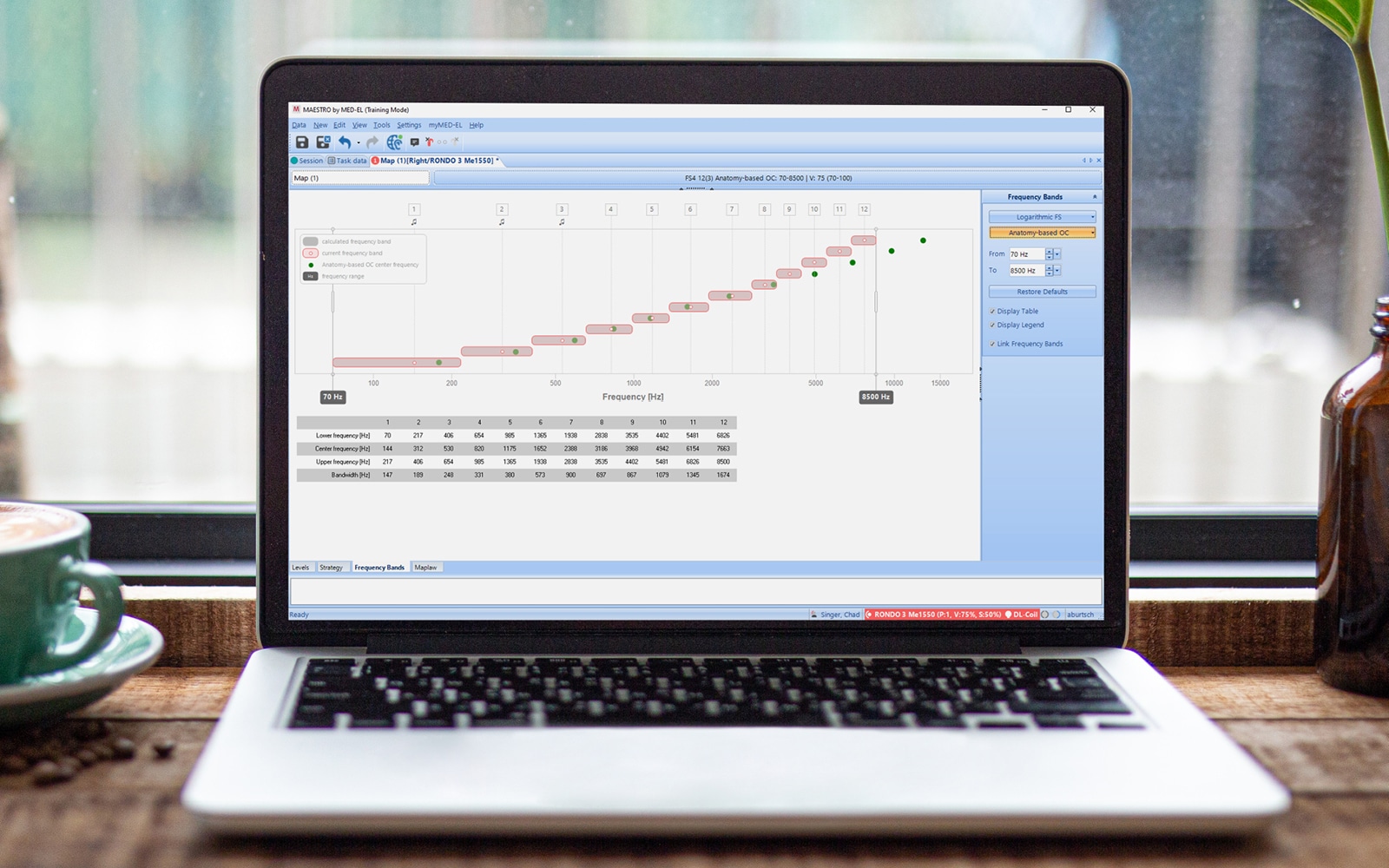
As previously shown, only MED-EL offers electrodes that are designed to stimulate beyond one and a half turns of the cochlea to make use of the cochlea’s full potential. In addition to longer electrodes, there is another way you can help your patients reach closer to natural hearing than ever before: with MAESTRO and place-based mapping using our anatomy-based fitting (ABF) tools. Similar to the points above, we have found better results with anatomy-based fitting with the ideal angular insertion depth. In other words, only when the electrode reaches one and a half turns angular insertion depth or beyond have we seen significant improvements thanks to anatomy-based fitting.
“ABF can be used to fit experienced bilateral cochlear implant users; it improves speech perception, especially in noise, and self-perceived sound quality. ABF fitting is a promising approach to match the input from both CIs, thereby providing access to binaural processing.”
Kurz et al., 2023
With anatomy-based fitting, you can easily fine-tune the frequency map to be closer to the natural map of each individual cochlea. By combining detailed imaging data from OTOPLAN with MAESTRO 10, you can quickly assign center frequencies based on the anatomical location of each electrode contact for MED-EL users with SONNET 2, SONNET 2 EAS, or RONDO 3 audio processors or later.
“The smaller the frequency-to-place mismatch, the better the initial speech perception in noise results of the CI recipients.”
Mertens et al., 2022
“Methods to minimize electric mismatches, such as place-based mapping procedures, may support better early speech recognition for [similar patient populations of EAS users].”
Dillon et al., 2023
Studies on this relatively new cochlear implant fitting approach have shown promising results for specific patient populations, such as bilateral cochlear implant recipients and electric-acoustic stimulation users. It may also help cochlear implant users benefit sooner after implantation.
And there’s exciting anatomy-based fitting news, especially for pediatric patients. One barrier to this place-based mapping approach has now been removed with the 2024 release of OTOPLAN, which allows electrode contacts to be located with a plain X-ray scan so the data can be exported for anatomy-based fitting.
9. Preserve Future Cochlear Health
A deaf ear is not a dead ear, and electrode design is crucial to preserve future cochlear health. Cochlear implant technology is rapidly advancing, and future technological advances may be able to leverage residual hearing and functioning structures to restore hearing perception. This makes implantation procedures that do minimal harm of utmost importance to ensure your patients can benefit from future technologies.
MED-EL FLEX electrodes have a proven record of structural preservation. This is evident both by examining hearing outcomes in MED-EL recipients with electric-acoustic stimulation and when considering the rate of successful scala tympani placements.
Because EAS users still have substantial residual hearing prior to being implanted with a FLEX electrode array, long-term post-operative residual hearing is a useful indication that the natural anatomy and physiology of the cochlea has been preserved. Several studies have shown that our flexible arrays enable a superior hearing preservation rate for combined electric-acoustic stimulation.Helbig, S., Adel, Y., Rader, T., Stoever, T., & Baumann, U. (2016). Long-term hearing preservation outcomes after cochlear implantation for electric-acoustic stimulation. Otol Neurotol. 37(9). https://doi.org/10.1097/MAO.0000000000001066[22]Manjaly, J.G., Nash, R., Ellis, W., Britz, A., Lavy, J.A., Shaida, A., Saeed, S.R., & Khalil, S.S., (2018). Hearing preservation with standard length electrodes in pediatric cochlear implantation. Otol Neurotol. 39(9). https://doi.org/10.1097/MAO.0000000000001917[23]US Food & Drug Administration. (2016). Premarket approval, MED-EL, MED-EL EAS System. P000025/S084. Premarket Approval (PMA)[24]Nassiri, A.M., Yawn, R.J., Gifford, R. H., Haynes, D.S., Roberts, J.B., Gilbane, M.S., Murfee, J., & Bennett, M.L. (2019). Intraoperative electrically evoked compound action potential (ECAP) measurements in traditional and hearing preservation cochlear implantation. J Am Acad Audiol. 30(10). 918¬–926. https://doi.org/10.3766/jaaa.18052[25]
Average Insertion Rate in the Scala Tympani
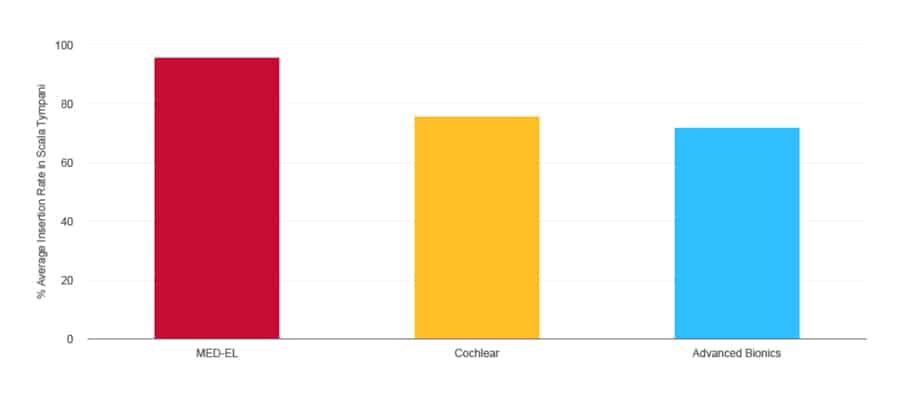
Literature Search Report (2020). MED-EL, Data on File. n=1,399
Scalar Deviation Rate
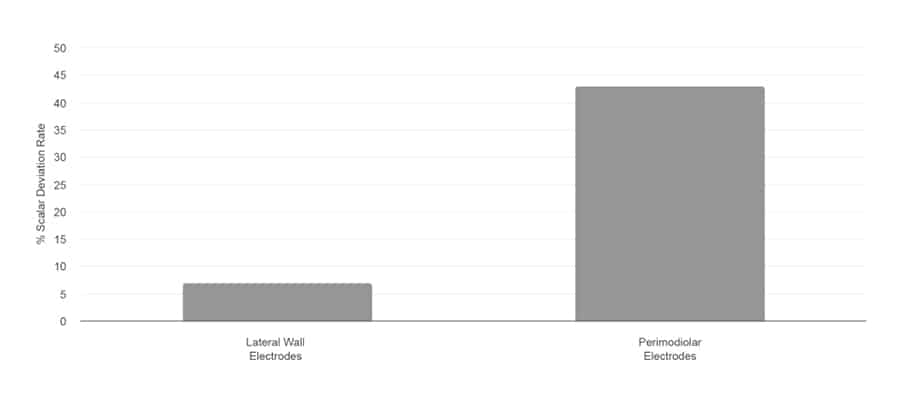
Jwair et al., 2020
“If one aims to minimize clinically relevant intracochlear trauma, lateral wall arrays would be the preferred option for cochlear implantation.”
Jwair et al., 2020
In addition, stiff electrode arrays are far more likely to penetrate and damage the delicate membranes between the scala tympani and scala vestibuli. In contrast, MED-EL’s flexible electrode arrays are proven to consistently enable scala tympani placement, with an average insertion rate of nearly 100%. This way, we can safely preserve those delicate structures and deliver better hearing performance to recipients.
And there’s also exciting news related to this topic: The 2024 release of OTOPLAN includes image fusion so you can get a view of the best information from each image modality (CT and MRI) combined. Notably, the image fusion feature can be used to confirm the postoperative placement of each electrode contact in the scala tympani with CT scan data.
Hearing Insights in Your Inbox
Get the latest research and resources to help people with every kind of hearing loss. Subscribe to the MED-EL Professionals Blog now.
Sign Up NowProvide Your Patients With the Closest to Natural Hearing
If you would like to know more about MED-EL’s FLEX electrodes, contact your local MED-EL team or visit our website to learn more about our electrode arrays.
References
-
[1]
Li, H., Schart-Morén, N., Rohani, S. A., Ladak, H. M., Rask-Andersen, H., & Agrawal, S. (2020). Synchrotron radiation-based reconstruction of the human spiral ganglion: Implications for cochlear implantation. Ear & Hearing, 41(1), 173–181. https://doi.org/10.1097/aud.0000000000000738
-
[2]
Li, H., Helpard, L., Ekeroot, J., Rohani, S. A., Zhu, N., Rask-Andersen, H., Ladak, H. M., & Agrawal, S. (2021). Three-dimensional tonotopic mapping of the human cochlea based on synchrotron radiation phase-contrast imaging. Scientific Reports, 11(1). https://doi.org/10.1038/s41598-021-83225-w
-
[3]
Helpard, L., Li, H., Rohani, S. A., Zhu, N., Rask-Andersen, H., Agrawal, S., & Ladak, H. M. (2021). An approach for individualized cochlear frequency mapping determined from 3D synchrotron radiation phase-contrast imaging. IEEE Transactions on Biomedical Engineering, 68(12), 3602–3611. https://doi.org/10.1109/tbme.2021.3080116
-
[4]
Dhanasingh, A. E., Rajan, G., & van de Heyning, P. (2020). Presence of the spiral ganglion cell bodies beyond the basal turn of the human cochlea. Cochlear Implants International, 21(3), 145–152. https://doi.org/10.1080/14670100.2019.1694226
-
[5]
Breitsprecher, T. M., Baumgartner, W. D., Brown, K., Dazert, S., Doyle, U., Dhanasingh, A., … & Weiss, N. M. (2023). Effect of Cochlear Implant Electrode Insertion Depth on Speech Perception Outcomes: A Systematic Review. Otology & Neurotology Open, 3(4), e045. https://doi.org/10.1097/ONO.0000000000000045
-
[6]
Weller, T., Timm, M. E., Lenarz, T., & Büchner, A. (2023). Cochlear coverage with lateral wall cochlear implant electrode arrays affects post-operative speech recognition. PLOS ONE, 18(7). https://doi.org/10.1371/journal.pone.0287450
-
[7]
Li, H., Schart-Moren, N., Rohani, S., A., Ladak, H., M., Rask-Andersen, A., & Agrawal, S. (2020). Synchrotron Radiation-Based Reconstruction of the Human Spiral Ganglion: Implications for Cochlear Implantation. Ear Hear. 41(1). https://doi.org/10.1097/AUD.0000000000000738
-
[8]
Landsberger, D. M., Svrakic, M., Roland, J. T., & Svirsky, M. (2015). The relationship between insertion angles, default frequency allocations, and spiral ganglion place pitch in cochlear implants. Ear & Hearing, 36(5). https://doi.org/10.1097/aud.0000000000000163
-
[9]
Landsberger, D. M., Vermeire, K., Claes, A., Van Rompaey, V., & Van de Heyning, P. (2016). Qualities of single electrode stimulation as a function of rate and place of stimulation with a cochlear implant. Ear & Hearing, 37(3). https://doi.org/10.1097/aud.0000000000000250
-
[10]
Li, H., Schart-Morén, N., Rohani, S. A., Ladak, H. M., Rask-Andersen, H., & Agrawal, S. (2019). Synchrotron radiation-based reconstruction of the human spiral ganglion: Implications for cochlear implantation. Ear & Hearing, 41(1), 173–181. https://doi.org/10.1097/aud.0000000000000738
-
[11]
McDermott, H., Sucher, C., & Simpson, A. (2009). Electro-Acoustic Stimulation: Acoustic and Electric Pitch Comparisons. Audiology and Neurotology, 14(Suppl. 1), 2–7. https://doi.org/10.1159/000206489
-
[12]
Roy, A. T., Penninger, R. T., Pearl, M. S., Wuerfel, W., Jiradejvong, P., Carver, C., Buechner, A., & Limb, C. J. (2016). Deeper cochlear implant electrode insertion angle improves detection of musical sound quality deterioration related to bass frequency removal. Otology & Neurotology, 37(2), 146–151. https://doi.org/10.1097/mao.0000000000000932
-
[13]
Schatzer, R., Vermeire, K., Visser, D., Krenmayr, A., Kals, M., Voormolen, M., Van de Heyning, P., & Zierhofer, C. (2014). Electric-acoustic pitch comparisons in single-sided-deaf cochlear implant users: Frequency-place functions and rate pitch. Hearing Research, 309, 26–35. https://doi.org/10.1016/j.heares.2013.11.003
-
[14]
Rader, T., Döge, J., Adel, Y., Weissgerber, T., & Baumann, U. (2016). Place dependent stimulation rates improve pitch perception in cochlear implantees with single-sided deafness. Hearing Research, 339, 94–103. https://doi.org/10.1016/j.heares.2016.06.013
-
[15]
Roy, A. T., Carver, C., Jiradejvong, P., & Limb, C. J. (2015). Musical Sound Quality in cochlear implant users. Ear & Hearing, 36(5), 582–590. https://doi.org/10.1097/aud.0000000000000170
-
[16]
Roy, A. T., Penninger, R. T., Pearl, M. S., Wuerfel, W., Jiradejvong, P., Carver, C., Buechner, A., & Limb, C. J. (2016). Deeper cochlear implant electrode insertion angle improves detection of musical sound quality deterioration related to bass frequency removal. Otology & Neurotology, 37(2), 146–151. https://doi.org/10.1097/mao.0000000000000932
-
[17]
Curtis, D. P., Baumann, A. N., & Jeyakumar, A. (2023). Variation in cochlear size: A systematic review. International Journal of Pediatric Otorhinolaryngology, 171, 111659. https://doi.org/10.1016/j.ijporl.2023.111659
-
[18]
Canfarotta, M. W., Dillon, M. T., Brown, K. D., Pillsbury, H. C., Dedmon, M. M., & O’Connell, B. P. (2021). Insertion depth and cochlear implant speech recognition outcomes: A Comparative Study of 28- and 31.5-mm lateral wall arrays. Otology & Neurotology, 43(2), 183–189. https://doi.org/10.1097/mao.0000000000003416
-
[19]
Dhanasingh, A. & Jolly, C. (2019). Review on cochlear implant electrode array tip fold-over and scalar deviation. J Otol. 14(3). 94-100. https://doi.org/10.1016/j.joto.2019.01.002
-
[20]
Micuda, A., Li, H., Rask‐Andersen, H., Ladak, H. M., & Agrawal, S. K. (2024). Morphologic analysis of the Scala tympani using synchrotron: Implications for cochlear implantation. The Laryngoscope. https://doi.org/10.1002/lary.31263
-
[21]
Koch, R. W., Ladak, H. M., Elfarnawany, M., & Agrawal, S. K. (2017). Measuring cochlear duct length – a historical analysis of methods and results. Journal of Otolaryngology – Head & Neck Surgery, 46(1). https://doi.org/10.1186/s40463-017-0194-2
-
[22]
Helbig, S., Adel, Y., Rader, T., Stoever, T., & Baumann, U. (2016). Long-term hearing preservation outcomes after cochlear implantation for electric-acoustic stimulation. Otol Neurotol. 37(9). https://doi.org/10.1097/MAO.0000000000001066
-
[23]
Manjaly, J.G., Nash, R., Ellis, W., Britz, A., Lavy, J.A., Shaida, A., Saeed, S.R., & Khalil, S.S., (2018). Hearing preservation with standard length electrodes in pediatric cochlear implantation. Otol Neurotol. 39(9). https://doi.org/10.1097/MAO.0000000000001917
-
[24]
US Food & Drug Administration. (2016). Premarket approval, MED-EL, MED-EL EAS System. P000025/S084. Premarket Approval (PMA)
-
[25]
Nassiri, A.M., Yawn, R.J., Gifford, R. H., Haynes, D.S., Roberts, J.B., Gilbane, M.S., Murfee, J., & Bennett, M.L. (2019). Intraoperative electrically evoked compound action potential (ECAP) measurements in traditional and hearing preservation cochlear implantation. J Am Acad Audiol. 30(10). 918¬–926. https://doi.org/10.3766/jaaa.18052
-
[26]
Hassepass, F., Bulla, S., Maier, W., Laszig, R., Arndt, S., Beck, R., Traser, L., & Aschendorff, A. (2014). The new Mid-Scala Electrode Array. Otology & Neurotology, 35(8), 1415–1420. https://doi.org/10.1097/mao.0000000000000412
-
[27]
Ketterer, M. C., Aschendorff, A., Arndt, S., Hassepass, F., Wesarg, T., Laszig, R., & Beck, R. (2018). The influence of cochlear morphology on the final electrode array position. European Archives of Oto-Rhino-Laryngology, 275(2), 385–394. https://doi.org/10.1007/s00405-017-4842-y
-
[28]
McJunkin, J. L., Durakovic, N., Herzog, J., & Buchman, C. A. (2018). Early outcomes with a slim, Modiolar Cochlear Implant Electrode Array. Otology & Neurotology, 39(1). https://doi.org/10.1097/mao.0000000000001652
-
[29]
Rader, T., Döge, J., Adel, Y., Weissgerber, T., & Baumann, U. (2016). Place dependent stimulation rates improve pitch perception in cochlear implantees with single-sided deafness. Hearing Research, 339, 94–103. https://doi.org/10.1016/j.heares.2016.06.013
-
[30]
Skarzynski, H., Matusiak, M., Furmanek, M., Pilka, A., Wlodarczyk, E., Oldak, M., & Skarzynski, P. H. (2018). Radiologic measurement of cochlea and hearing preservation rate using Slim Straight Electrode (CI422) and round window approach. Acta Otorhinolaryngologica Italica, 38(5), 468–475. https://doi.org/10.14639/0392-100x-1579
-
[31]
Dutrieux, N., Quatre, R., Péan, V., & Schmerber, S. (2021). Correlation between cochlear length, insertion angle, and tonotopic mismatch for Med-El Flex28 electrode arrays. Otology & Neurotology, 43(1), 48–55. https://doi.org/10.1097/mao.0000000000003337
-
[32]
Kurz, A., Herrmann, D., Hagen, R., & Rak, K. (2023). Using Anatomy-Based Fitting to Reduce Frequency-to-Place Mismatch in Experienced Bilateral Cochlear Implant Users: A Promising Concept. Journal of Personalized Medicine, 13(7), 1109. https://doi.org/10.3390/jpm13071109
-
[33]
Mertens, G., Van de Heyning, P., Vanderveken, O. et al. The smaller the frequency-to-place mismatch the better the hearing outcomes in cochlear implant recipients?. Eur Arch Otorhinolaryngol 279, 1875–1883 (2022). https://doi.org/10.1007/s00405-021-06899-y
-
[34]
Dillon, M. T., Canfarotta, M. W., Buss, E., Rooth, M. A., Richter, M. E., Overton, A. B., Roth, N. E., Dillon, S. M., Raymond, J. H., Young, A., Pearson, A. C., Davis, A. G., Dedmon, M. M., Brown, K. D., & O’Connell, B. P. (2023). Influence of Electric Frequency-to-Place Mismatches on the Early Speech Recognition Outcomes for Electric–Acoustic Stimulation Users. American Journal of Audiology, 32(1), 251–260. https://doi.org/10.1044/2022_aja-21-00254
-
[35]
Schatzer, R., Vermeire, K., Visser, D., Krenmayr, A., Kals, M., Voormolen, M., Van de Heyning, P., & Zierhofer, C. (2014) Electric-acoustic pitch comparisons in single-sided-deaf cochlear implant users: frequency-place functions and rate pitch. Hear Res., 309, 26–35. https://doi.org/10.1016/j.heares.2013.11.003
-
[36]
Dhanasingh, A., Nielsen, S. B., Beal, F., Schilp, S., Hessler, R., Jolly, C., & Hochmair, I. (2024). Cochlear implant electrode design for safe and effective treatment. Frontiers in Neurology, 15, 1348439. https://doi.org/10.3389/fneur.2024.1348439
References
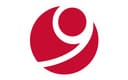
MED-EL
Was this article helpful?
Thanks for your feedback.
Sign up for newsletter below for more.
Thanks for your feedback.
Please leave your message below.
CTA Form Success Message
Send us a message
Field is required
John Doe
Field is required
name@mail.com
Field is required
What do you think?
The content on this website is for general informational purposes only and should not be taken as medical advice. Please contact your doctor or hearing specialist to learn what type of hearing solution is suitable for your specific needs. Not all products, features, or indications shown are approved in all countries.
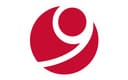
MED-EL
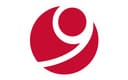
MED-EL