
MED-EL
Published May 10, 2017
Why Long Electrode Arrays: More Natural Tonotopic Coding
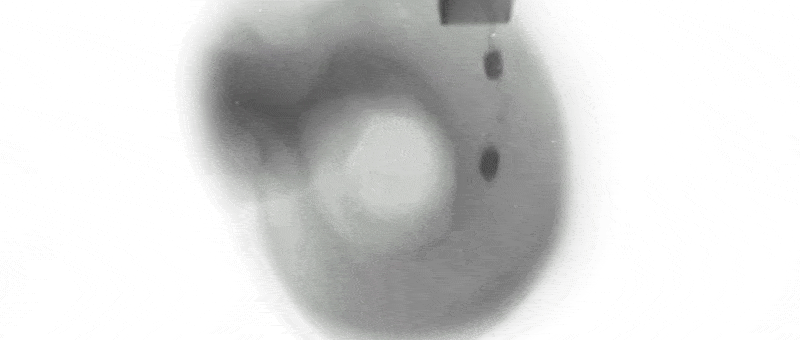
The human cochlea has remarkable arrangement. Along two and a half turns, natural frequency response is intricately ordered in a descending logarithmic scale. This tonotopic frequency response along the whole cochlea allows frequency mapping along a clear, logical path—the Greenwood function.1
With the Greenwood function, you can determine the natural tonotopic frequency of any point along the basilar membrane. You’ll only need to know the cochlear duct length, measured from center of the round window to the helicotrema, which corresponds to the length of the Organ of Corti.
Short Electrode Arrays = Less Coverage
In humans, the average cochlear duct length (CDL) has been measured as approximately 31.5 mm, though it can range up to 35 mm.2,3 Interestingly, the basal turn alone can account for the first ~20 mm of the total cochlear duct length.2,3,4
Say you take a common pre-curved or straight electrode array, designed for a 20 mm insertion depth. These arrays are often marketed as “full-length”, but with only 14–19.1 mm of active stimulation length, only a single turn can be stimulated for most cochleae.3,5,6 These arrays may be inserted deeper, but it would limit coverage of high frequencies in the first turn.5
So, where would a 20 mm insertion reach along the tonotopic map of the basilar membrane?
- In an average cochlea (31.5 mm CDL), a 20 mm insertion would reach the 820 Hz tonotopic location.1,2,3
- In a slightly longer cochlea, (32.5 mm CDL), 20 mm would correspond to the 917 Hz location.1,2,3
- Even in a smaller-than-average cochlea (29.5 mm CDL), 20 mm would only reach the 640 Hz location.1,2,3
Out of Reach
So with these single-turn arrays, even the most apical electrode may likely only reach roughly the 600–800 Hz position on the natural tonotopic map.1,2,3,7 If an electrode array can’t reach the apical region in the second turn, low-to-mid pitches cannot be naturally mapped according to the Greenwood function.1,5,7
Now, do fundamental frequencies lower than 600–800 Hz really matter? Let’s take a look at a few examples of what falls below 700 Hz.
It’s not just the drums or bass that’d be missing. For reference, Middle C (C4) on a piano is 261.63 Hz, and the highest-pitch open string on a violin is 659 Hz (E5). A standard guitar tuning doesn’t reach much higher than 659 Hz (E5 up on the 12th fret). On the other hand, above 700 Hz you’d still find most of the notes from a piccolo.
This high-pitch tuning doesn’t just come up short at the concert hall. In everyday conversation, the fundamental frequencies and lower harmonics of voice are much lower than 700 Hz.8,9
- Male voice: F0 ~120 Hz, F1 ~240 Hz, F2 ~360 Hz, F3 ~480 Hz, F4 ~600 Hz
- Female voice: F0 ~200 Hz F1 ~400 Hz, F2 ~600 Hz
The natural tonotopic coding of the cochlea is like the tuning of the keys on a piano. Without a long electrode array to better cover the cochlea, most of the notes are simply out of reach, so lower pitches have to be transposed onto a higher octave.
Place-Pitch Mismatch
Lower frequencies can be mapped on single-turn arrays, but there will likely be a significant place-pitch mismatch.1,5,7,10 This unnaturally skewed pitch mapping may shift low frequencies up by roughly two octaves, so sound quality can be distinctly affected.
With short arrays and single-turn insertions, outcomes can often be less than ideal.
- Experienced users have described the sound as “robotic”, “tinny”, “echoey”, or “mechanical” 11
- Reduced speech understanding in quiet and in noise 12,13,14,15
- Neural adaptation can take years for even a single octave correction 7
- Limited improvement in speech perception over time 13
Complete Cochlear Coverage
On the other hand, long electrode arrays (28 mm, 31.5 mm, or 34 mm) enable electrode coverage across the first and second turn of the cochlea. This allows tonotopic stimulation of mid-to-low frequencies that’s place-pitch matched to the Greenwood function down to approximately 100 Hz.5,10,16
Better Coverage, Better Outcomes
Cochlear implants aren’t able to restore natural hearing—but we believe they should offer a closer match when possible. When it comes to more natural sound quality, this accurate place-pitch match is the right place to start.16
What benefits can long electrode arrays provide?
- “Vocals much more clear and resonant” and “Deeper, more resonant, more natural sound” 11
- Significantly better speech understanding in quiet and noise 13,15
- More natural frequency mapping 16,17
- Significant improvement in speech understanding in a short time 13,18
Hint: “An unparalleled restoration of tonotopic pitch perception”—Rader et al. (2016)16
Subscribe now to make sure you don’t miss part two!
*Not all products, features, or indications shown are available in all areas. Please contact your local MED-EL representative for more information.
References:
- Greenwood, D.D. (1990) A cochlear frequency-position function for several species–29 years later. J Acoust Soc Am., 87(6), 2592–2605.
- Hardy, M. (1938). The length of the organ of Corti in man. J. Anat., 62, 291–311.
- Ni, Y. Dai, P., Dai, C., & Li, H.. (2017) Cochlear implant-related three-dimensional characteristics determined by micro-computed tomography reconstruction. Clin Anat., 30(1), 39–43.
- Adunka, O., Unkelbach, M.H., Mack, M.G., Radeloff, A., & Gstoettner, W. (2005) Predicting basal cochlear length for electric-acoustic stimulation. Arch Otolaryngol Head Neck Surg., 131(6), 488–492.
- Landsberger, D.M., Svrakic, M., Roland, J.T. Jr., & Svirsky, M. (2015). The relationship between insertion angles, default frequency allocations, and spiral ganglion place pitch in cochlear implants. Ear Hear., 36(5), 207–213.
- Radeloff, A. Mack, M., Baghi, M., Gstoettner, W.K., & Adunka, O. (2008). Variance of angular insertion depths in free-fitting and perimodiolar cochlear implant electrodes. Otol Neurotol., 29(2), 131–136.
- McDermott, H., Sucher, C., & Simpson, A. (2009) Electro-acoustic stimulation. Acoustic and electric pitch comparisons. Audiol Neurootol., 14(1), 2–7.
- Pepiot, E. (2014). Male and female speech: a study of mean f0, f0 range, phonation type and speech rate in Parisian French and American English speakers. Speech Prosody, 7, 305–309.
- Eriksson, A., & Traunmueller, H. (1994). The frequency range of the voice fundamental in the speech of male and female adults.
- Prentiss, S., Staecker, H., & Wolford, B. (2014). Ipsilateral acoustic electric pitch matching: a case study of cochlear implantation in an up-sloping hearing loss with preserved hearing across multiple frequencies. Cochlear Implants Int., 15(3), 161–165.
- Harris, R.L., Gibson, W.P. Johnson, M., Brew, J., Bray, M., & Psarros, C. (2011) Intra-individual assessment of speech and music perception in cochlear implant users with contralateral Cochlear and MED-EL systems. Acta Otolaryngol., 131(12), 1270–1278.
- Arnoldner, C., Riss, D., Baumgartner, W.D., Kaider, A., & Hamzavi, J.S. (2007). Cochlear implant channel separation and its influence on speech perception–implications for a new electrode design. Audiol Neurootol., 12(5), 313–324.
- Buchman, C.A., Dillon, M.T., King, E.R., Adunka, M.C., Adunka, O.F., & Pillsbury, H.C. (2014). Influence of cochlear implant insertion depth on performance: a prospective randomized trial. Otol Neurotol., 35(10), 1773–1779.
- O’Connell, B.P., Cakir, A., Hunter, J.B., Francis, D.O., Noble, J.H., Labadie, R.F., Zuniga, G., Dawant, B.M., Rivas, A., & Wanna, G.B. (2016) Electrode location and angular insertion depth are predictors of audiologic outcomes in cochlear implantation. Otol Neurotol. 37(8):1016–1023.
- O’Connell, B.P., Hunter, J.B., Haynes, D.S., Holder, J.T., Dedmon, M.M., Noble, J.H., Dawant, B.M., & Wanna, G.B. (2017) Insertion depth impacts speech perception and hearing preservation for lateral wall electrodes. Laryngoscope. Epub ahead of print.
- Rader, T., Döge, J., Adel, Y., Weissgerber, T., & Baumann, U. (2016). Place dependent stimulation rates improve pitch perception in cochlear implantees with single-sided deafness. Hear Res., 339, 94–103.
- Vermeire, K., Landsberger, D.M., Van de Heyning, P.H., Voormolen, M., Kleine Punte, A., Schatzer, R., & Zierhofer, C. (2015) Frequency-place map for electrical stimulation in cochlear implants: Change over time. Hear Res., 326,8–14.
- Helbig, S., Helbig, M., Leinung, M., Stöver, T., Baumann, U., & Rader, T. (2015). Hearing preservation and improved speech perception with a flexible 28-mm electrode. Otol Neurotol. 2015 Jan;36(1):34-42.
MED-EL
Was this article helpful?
Thanks for your feedback.
Sign up for newsletter below for more.
Thanks for your feedback.
Please leave your message below.
CTA Form Success Message
Send us a message
Field is required
John Doe
Field is required
name@mail.com
Field is required
What do you think?
The content on this website is for general informational purposes only and should not be taken as medical advice. Please contact your doctor or hearing specialist to learn what type of hearing solution is suitable for your specific needs. Not all products, features, or indications shown are approved in all countries.

MED-EL