
MED-EL
Published Sep 07, 2021
What Does a Cochlear Implant Sound Like? Reaching Closest to Natural Hearing With MED-EL
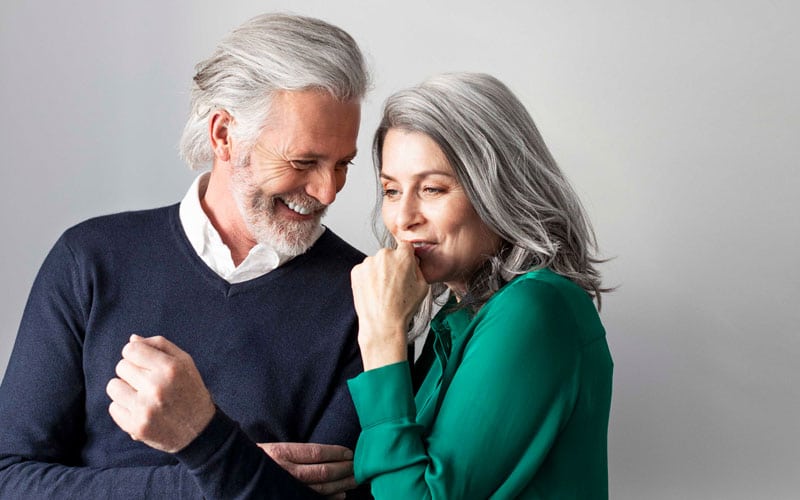
As a clinician, you probably have heard these questions many times: What does a cochlear implant sound like? Is it robotic? Will I recognize voices? Will I be able to listen to music? How long does it take to get used to?
These are all perfectly natural questions—after all, your patients are choosing a cochlear implant to hear.
You might have come across cochlear implant sound simulations online. These popular videos or sound samples are often simulations made using a vocoder, which is an audio tool that can be used to artificially synthesize voices.
It is very important to understand that a vocoder “cochlear implant simulation” is not at all an accurate representation of what a cochlear implant sounds like. These simulations sound harsh, robotic, and uncomfortable to listen to, and do not at all match real user experience.1,2
If you Google ‘cochlear implant simulation’, you’ll find noise-band vocoders. I’m pleased to say that a cochlear implant does not sound like a vocoder. It would be painful if you had to listen to something like a vocoder all your life. No, it doesn’t sound like a noise vocoder or a sine vocoder, thankfully. –Prof. Michael Dorman
Thankfully, there has been extensive research on this topic. Cochlear implant recipients who have single-sided deafness can directly compare the sounds of their cochlear implant to the same sound played to their natural hearing ear.1–9
“No! . . .No, no, no. . .No!” –A cochlear implant user with single-sided deafness comparing vocoder simulations in their natural hearing ear with their implanted ear.
The problem is that these inaccurate representations of cochlear implants can really discourage candidates from moving forward and experiencing all the benefits of cochlear implants.
“I was hesitant to pursue a cochlear implant for myself. Why? It’s those cochlear implants simulations that are available online. So you go and you listen to ‘what speech sounds like with a cochlear implant’ and ‘this is what music sounds like with a cochlear implant’. I didn’t want that. It sounds terrible.” . . . “When they play those pseudoscientific clips about music and speech with a cochlear implant, I’ve complained to them, “It’s going to discourage people from getting a cochlear implant.” –Two cochlear implant recipients discussing how inaccurate representations can discourage candidates.
However, there are certainly a wide range of experiences with cochlear implants, and this is especially true for sound quality. You likely have heard users describe their implants as sounding “robotic”, “distorted”, “tinny”, or “squeaky”, while other users describe their hearing as surprisingly natural and pleasant.
So what is causing this difference, and can we support better outcomes?
Often, candidates and clinicians are inclined to believe that all cochlear implants are more or less the same. However, it’s absolutely essential to understand that not all cochlear implants are created equal. Extensive research has shown different technology = significantly different outcomes.1–24
“More robotic, tinny and echoey. Cannot differentiate different individuals’ voices as well because more metallic sound. Sounds like a distorted microphone.”—Cochlear implant recipient describing sound quality through their Cochlear-brand implant with a short electrode array.19
“Immediately loved the sound. . .vocals much more clear and resonant. More natural sound. Less fatigue from MED-EL because guessing less.” Same recipient describing their other ear implanted with a long MED-EL electrode array.19
Today, we’re looking at the fundamental science of cochlear implant sound quality and why implant design is such an important factor in hearing outcomes.
- Why tonotopic pitch-place matching is so essential to sound quality.
- Controllable factors, such as device choice and electrode placement, that can have a significant impact on cochlear implant sound quality.
- What MED-EL CI users have to say about the sound quality of their implants in everyday life.
This deep-dive is made for professionals who are familiar with cochlear implants. If you’d like a version of this information that’s a little easier to follow, make sure to check out our Closest to Natural Hearing page for cochlear implant candidates and their families. There are interactive learning tools to help people understand the fundamentals of sound, hearing, and cochlear implants, together with a comprehensive explanation of cochlear implant sound quality and benefits of more natural hearing.
Designed to Follow Nature
What does a cochlear implant sound like? Well, what if we ask ourselves “what should a cochlear implant sound like”? If your patients are choosing a cochlear implant to hear, how can we help give them the best possible listening experience?
Our sense of hearing is not just the ability to detect sounds or understand words. Hearing is all the sounds of life: the joy of laughter, the crackle of leaves, and the emotional lift of music. Hearing goes so much beyond just understanding a sound. Our natural hearing has such a powerful and emotional connection to the human experience.
This is why at MED-EL, our philosophy is to support the closest to natural hearing with our cochlear implants. For more than 30 years we have designed our cochlear implants to follow the design of nature as closely as possible. Our one-of-a-kind technologies each drive us a step closer to natural hearing, and together, they set the hearing experience with a MED-EL cochlear implant apart from any other cochlear implant.1–25
More natural hearing doesn’t just mean a more enjoyable sound quality. Enabling more natural sound input can help the brain to understand and comprehend complex sounds more easily. This can make it easier to actively participate in group conversations and be especially useful in challenging environments like noisy restaurants or lively playgrounds.10,11,14,24
“My ideal cochlear implant would be one that the hearing experience is so natural that a recipient does not ‘realize’ they have an implant.” Dr. Peter Nopp—Director of Sound Coding Research, MED-EL
Natural Foundation
So how can we achieve a closer to natural hearing experience with a cochlear implant? First, we need to look at how a cochlear implant interacts with the natural auditory system.
As you know, sensorineural hearing loss is caused when the sensory hair cells in the cochlea do not function properly. However, it’s important to understand that these hair cells are just one step in the natural hearing process—and in sensorineural hearing loss, the rest of the natural neural structures are more or less intact.
Cochlear implants are not like a prosthetic limb that replaces the natural structures—instead, they are designed to work precisely together with the intricate neural pathways of natural hearing.
“A deaf ear is not a dead ear! The human auditory nerve function persists after hair cell loss and deafness; a blessing for the deaf and implanted.” Prof. Helge Rask-Andersen
It may sound odd, but even in profound sensorineural hearing loss, the rest of the delicate structures of the cochlea can be healthy. There is an incredibly intricate network of nerve cells that are just waiting to be reactivated. If a light switch is broken, you don’t need to replace all the wiring in the walls—you just need to replace the switch.
If a cochlear implant could stimulate the natural nerve structures with the same precision as in normal hearing, then theoretically the brain would have no way of knowing the difference between natural hearing and a cochlear implant, because the nerve signals would be identical.
In natural hearing, hair cells in the organ of Corti (OC) send nerve signals through the spiral ganglion (SG) and along the auditory nerve to the brain. A cochlear implant targets these same nerve structures organ of Corti and spiral ganglion.
What does this mean for the sound quality of a cochlear implant? The answer is clear—sound quality is closely connected to how closely you can follow nature when you stimulate that cochlea.1–25
This is why the electrode array is such an essential factor in sound quality. The electrode array is the intricate bridge between technology and nature—every single bit of sound information from the CI system has to flow through that delicate interface to the natural hearing pathways.
Even with the most advanced audio processor, you’ll hit a “quality bottleneck” if the electrode array isn’t made to provide a closer match to natural hearing. Think about streaming music—a direct connection to the audio processor is great, but it doesn’t mean much if that music is distorted by the limitations of the sound coding and electrode array.
The truth is that every single sound your patients will hear through a cochlear implant goes through the tiny electrode array that is implanted in their cochlea.
Audio processors are replaced every few years, but the implant is a decision for many, many years. Patients might upgrade their audio processor as often as they upgrade their mobile phone, but we have many recipients who have been using their implant for 25+ years. This is why having the right implant and the right electrode array is such an essential factor in cochlear implant sound quality.
Sound Quality: Natural Loudness and Frequency
With this in mind, let’s take a look at the nature of sound, so that we can see how to create more natural sound quality. There are two key elements of sound to consider for CIs: loudness (in dB) and frequency (in Hz).
Loudness
In cochlear implants, loudness is basically determined by the dynamic range of a CI—how much of a perceived volume difference you can represent between low levels and high levels. Natural speech and music have a wide dynamic range, so this is an important aspect of sound quality.
Many CI systems have very high or even infinite compression after ~70dB. This unnatural compression means that at a cocktail party on a runway, a person talking to you and a jet engine next to you would be presented at the same stimulation level. Or in everyday life, nearby traffic could muffle out a person shouting to you. A limited dynamic range can make it difficult to follow the natural loudness envelope of sound.25
To enable more natural loudness perception, MED-EL CIs use a natural 3:1 compression ratio that is designed to match the natural compression ratio of the basilar membrane. This means sounds can get noticeably louder, from ~30dB all the way up to ~110 dB. Our dual-loop Automatic Gain Control also instantly and seamlessly adapts to help make sure quiet sounds are audible and loud sounds are comfortable.
Loudness is an important aspect of sound quality, but a quiet dog barking will still sound like a dog. So what is causing the “tinny” or “squeaky” distortions that are reported by cochlear implant users?
Frequency
This is where the frequency or pitch of sound is so important. Frequency is a key part of what gives a natural sound its “character”. On a piano, each key sounds different because they each play a unique pitch.
If you alter the frequency too much, a sound will sound very unnatural. For example, shifting frequencies up can make them sound “thin”, “tinny” or “squeaky”—a common complaint from cochlear implant users.1,2,5,9,19
This is why the main question in sound quality is “how accurately can the CI represent the natural frequency or pitch of a sound?”
As we saw, a cochlear implant is designed to connect to the natural neural hearing pathway in the cochlea. So it’s only logical that a cochlear implant can sound more natural if it can interact more accurately with the natural design of our cochlea.4,7,10,11,12,13,14,15
Natural Hearing: The Right Place
In natural hearing, each specific frequency of sound activates a corresponding specific group of hair cells at a specific place in your cochlea. This is called tonotopic (pitch-place) coding, and it’s just like a piano keyboard laid out all along the cochlea, from the beginning to the tip.13
This means a high-pitched bird chirp activates hair cells at the beginning of your cochlea, while a lower-pitched male voice activates hair cells deeper in, near the tip of your cochlea.
Cochleae come in an incredible range of different shapes and sizes, but the rotational angle to frequency place-pitch map is very reliable and consistent across typical cochleae. This means that the natural center frequency of the hair cells at 1 turn in the spiral (360°) is always ~940Hz, even in different sized cochlea.
In fact, this place-pitch matching is so precise and reliable that if you activate a certain region of hair cells, your brain will automatically perceive it as that specific pitch. Just like a piano keyboard, pressing the same key always plays the same sound. This is how your brain understands the frequency of sounds—pitch perception is determined by which hair cells are being activated.
This tonotopic frequency map is so central to our natural hearing that it is maintained all the way to the auditory centers of the brain. You can map specific frequencies to their natural pitch-place in the brain, so changing the tonotopic map of the cochlea could also involve considerable remapping of the brain.
Cochlear Implants: The Right Place
We know that the hair cells are arranged in order of frequency and go almost all the way to the tip of the cochlea. And we know that a cochlear implant electrode array can’t stimulate nerve fibers that it can’t reach. What does this mean?
To provide an accurate perception of a sound, you should stimulate that sound’s frequency in the correct natural place in the cochlea.3–15
A cochlear implant electrode array has to be long enough to cover the whole cochlea, so that it can stimulate a full natural range of sound frequencies. You can’t accurately stimulate frequencies if the electrode array doesn’t cover that part of the cochlea—just like trying to play piano keys you can’t reach.
This is why long electrode arrays are the key to a more natural sound quality with a cochlear implant. Extensive research has shown that this accurate place-pitch matching is the only reliable way to provide a more accurate pitch perception with a cochlear implant.1–20
In the cochlea, the nerve dendrites connected to the hair cells go up to about 720°, or two full turns. This is why a long electrode array is essential—you can’t stimulate nerve structures you can’t reach.
Long Arrays: Complete Cochlear Coverage
For more natural hearing, a reasonable goal is to provide an accurate pitch match to the range of frequencies picked up by the audio processor. In a current MED-EL audio processor, this range is 70Hz to 8,500 Hz, which also correlates well to the frequencies on an audiogram (125–8,000 Hz).
So to reach that target low frequency range, you’d want an electrode array with the most apical (tip) contact that reaches about 630°–720° angular insertion depth to provide complete cochlear coverage. As we’ll see a bit later, rate coding can help fine-tune the low-frequency alignment in the second turn of the cochlea.4,13,20
In the average-sized cochlea, this would require a free-fitting lateral wall array that is approximately 28–31.5 mm long. Small cochleae would need a slightly shorter array for the same coverage, and a larger cochlea would need a longer array.13,17,20
To enable complete cochlear coverage for all different size cochleae, MED-EL offers the most comprehensive portfolio of electrode arrays, include 5 different lengths of our industry-leading FLEX arrays.
Our unique FLEX-Tip technology and free-fitting arrays make it possible to safely insert our electrode arrays up to 720°, offering an optimal level of cochlear coverage that no other company can match. With MED-EL’s long arrays, you can achieve complete cochlear coverage for each individual cochlea, providing the foundation for the closest to natural hearing.
Short Arrays: Missing Coverage
What about pre-curved arrays? These 15–23 mm arrays are commonly used by other CI companies, and are often marketed as “sitting closer to the hearing nerve”. They’re also often promoted as “full-length”, which could only be considered true in the shortest possible cochleae.7,16,20
In the cochlea, your auditory nerve is shaped a bit like a Christmas tree: the tips of the branches are individual peripheral nerve fibers that reach your hair cells, while closer to the trunk of the tree is the spiral ganglion, which continues on to the auditory nerve. Pre-curved arrays wrap closer to the center of the cochlea, so they are more likely to stimulate the spiral ganglion than the tips of the fibers.
Although this feature is often marketed as a benefit, it is actually more of a necessity for the basic function of the design. If these pre-curved arrays are not close enough to the spiral ganglion, there can be a significant loss in performance. The electrode contacts are closely spaced, so there can be issues with overlapping stimulation if they’re not close enough to the modiolus of the cochlea.21
Most importantly, pitch perception has nothing to do with how close the electrode is to the center of the cochlea. In practical terms, perimodiolar placement has no advantage for place-pitch matching, because the organ of Corti (OC) and spiral ganglion (SG) have nearly identical pitch-place mapping up to about 650°–700°, and perimodiolar arrays are never able to reach this deeply.13,16
Natural frequency map: For short arrays that only reach 360°–450°, stimulating the spiral ganglion (orange) doesn’t effectively reach frequencies lower than stimulating the hair cells in the organ of Corti (green).
Due to design limitations, perimodiolar arrays and stiff mid-scala arrays cannot safely reach much beyond the first turn of the cochlea, so those arrays generally stop at 360°–450°. With these stiff arrays, deeper insertion would likely cause significant trauma to the cochlea.16
Many electrode arrays only cover the basal turn of the cochlea, which means a large portion of the natural hearing nerve structures can’t be reached.
This is one of the most significant limitations of this design philosophy: Because pre-curved arrays cannot reach much beyond the first turn of the cochlea, they can only cover approximately half the relevant natural frequency map.7,13,16
Incidentally, these short pre-curved arrays are often marketed as offering the ideal electrode length to balance “coverage and structure preservation”, even though ultra-flexible long MED-EL arrays have consistently higher rates of structure and hearing preservation.
Even with less rigid pre-curved arrays, the tightening curvature of the cochlea makes it essentially impossible for a pre-curved array to hug the modiolus beyond the first turn without encountering near-automatic tip fold-over.26
There are also claims that these electrodes are “on the inside track” of the cochlea, so they can stimulate just as much as longer arrays. This is not at all supported by clinical evidence—post-operative imaging consistently shows that these arrays only cover the first turn of the cochlea (360°–450°).7,16,20,26
This means being “closer to the hearing nerve” certainly does not mean “more natural sound quality”. The nerves in your elbow are closer to your brain than your fingertips, but of course your fingertips have more precision. And if you are missing any electrode coverage of half of those natural hearing nerve structures, can you honestly call that “closer to the hearing nerve”?
This is why electrode length is the essential factor when it comes to matching the natural tonotopic map of the cochlea.
Place-Pitch Mismatch: Distorted Sound
It is important to note that short electrode arrays can generally provide reasonable speech understanding, especially in quiet settings, which is a benefit for users. Being able to understand speech is key to communication, so speech understanding is often used as a mark of success.
However, it’s essential to understand that “intelligible” on a speech test does not necessarily mean “natural” or “enjoyable”. With a short array, the quality of the sound can often be greatly altered, and this can mean a user’s listening experience may be functional, but not necessarily enjoyable.1,2,9,15,18,19
A significant upshift in frequencies often causes sounds to be “tinny”, meaning that they sound thin, metallic, and lacking depth. This is because the lower frequencies that created the depth and body of sounds are lost. And this distortion can also make it difficult to pick out more subtle frequency cues that are helpful for speech understanding in noise.10,11,12,13,14,15,19
Generally speaking, these shorter arrays that can only stimulate the first turn of the cochlea, which would mean only middle-to-high frequencies can be stimulated in their natural place, usually starting at around 800 Hz.20
If a CI just ignored all the frequencies it could not reach, those users would be missing so many sounds in everyday life. For example, the fundamentals of male voice are at ~120 Hz and female voice at ~200 Hz. And on a standard piano keyboard, more than 2/3 of the keys are below 800 Hz. So instead of ignoring all these frequencies, these systems shift the missing sounds into the part of the cochlea they can reach.
This significant frequency compression and transposition can have an obvious impact on sound quality: the ~120Hz fundamentals of the male voice would be stimulated at the much higher pitched place of ~800 Hz, and therefore perceived as closer to 800 Hz. This is quite a bit higher than the ~200Hz fundamentals or even the first few harmonics of a female voice, and these distorted frequencies can make it difficult to recognize the gender of a speaker.28
And of course, this frequency mismatch on short arrays can have a serious effect on overall hearing experience: 1,2,5,9,10,14,15,18,19
- Unnatural voice perception
- Tinny or robotic sound quality
- Difficulty enjoying music
- Limited speech understanding in noise
With so many of these short array implants currently in use, it’s not surprising that the public perception of CIs often leans towards unnatural sound quality.
But it doesn’t have to be this way. There are many patient factors that can influence outcomes with a cochlear implant, but the electrode array is fundamentally a controllable device factor for each candidate: Different designs = significantly different outcomes.
Electrode Channels: 22 vs. 12
We’ve seen why electrode array length is so important, but you’ve probably heard the argument “22 active contacts support better performance”. This certainly seems like a logical argument because an electrode contact is where the implant stimulates the cochlea.
However, there are two things to consider. Is the implant system limited to physical electrode contacts or is the implant advanced enough to create virtual channels between two contacts, and how much of the cochlea is covered by those contacts?
MED-EL is the only company that combines precisely coordinated stimulation between each electrode contact together with long arrays to provide up to 250 virtual channels. By covering the whole cochlea and utilizing up to 250 virtual channels, MED-EL implants offer a sound quality that no other implant can match.5,12,15,22
Virtual Channels: 250 vs. 22
More advanced CI systems can use parallel or coordinated sequential stimulation on two or more contacts. By precisely coordinating the stimulation between two adjacent electrode contacts, it’s possible to create additional “virtual” electrode channels, which act like additional electrode contacts between those two real physical electrode contacts.
With bell-shaped frequency filter bands, our unique FineHearing technology can create multiple virtual channels between each pair of our optimally spaced physical contacts. This means the need for numerous physical electrode contacts is effectively replaced by these virtual electrode contacts.
Our world-class Ti100 implant electronics use independent current sources for every channel, meaning each active electrode contact has its own high-speed precision controller. With this powerful implant hardware, our default sound coding strategy is able to utilize both parallel and rapid coordinated sequential stimulation.
This makes it possible for a MED-EL implant with 12 active channels to stimulate up to 250 unique virtual channels or spectral bands. You can think of this like being able to play all the keys on a piano, even though you only have 10 fingers. If you can reach both ends, you can reach the spaces in between. This powerful technology is part of our default FineHearing sound coding.
On the other hand, if an implant has 22 contacts, but isn’t designed to create virtual channels, this may be more like playing only 22 piano keys. And with current “n of m” sound coding strategies on these implants, it’s only 8 of 22 electrodes active at a given moment, so “most active electrodes” isn’t really accurate. These implants also require passive dissipation of DC current, rather than active balancing, so stimulation rates are limited. In hardware terms, this is often referred to as “limited headroom”, because there’s not so much capacity to push the hardware further with faster, more complex coding in the future.27
It’s certainly possible to provide acceptable outcomes with 22 closely spaced contacts, but this design couldn’t be called more advanced just because it has more electrode contacts. If contacts are too closely spaced, there is less room for virtual channels and a higher potential for undesired channel interaction through overlapping stimulation. More electrode contacts on a short array can also make the array stiffer, and more likely to damage the natural structures of the cochlea.16,21
But most importantly, it matters where those electrode contacts are. If you have 16 or 22 physical electrode channels, but they only cover the first half of the cochlea, then half of the natural frequency range will be missing. Even if you theoretically put 1,000 electrode contacts on an implant, but the array only covered half the cochlea, the result is the same. The unnatural “tinny” sound is caused by forcing frequencies upwards, not by limiting the number of electrode channels.5,6,7,13,19,21
Closest Match From the Beginning
What about adaptation? Don’t CI users adapt to pitch mismatches over time? A common argument is that length of the electrode array doesn’t matter because our brain will just adapt to correct any mismatch. This theory has been widely promoted, but numerous studies have shown that adaptation is really not a reliably effective method to correct significant tonotopic mismatch.9,10,11,18,19
It’s true that every CI will require some degree of adaptation and accommodation as the brain learns to process this new input. However, how much and how well each CI user adapts depends on many factors. And any changes certainly take time and effort, so minimizing the need for adaptation would be a benefit.9,10,11,15,18,19
The natural tonotopic organization of the human cochlea is incredibly robust. Forcing this system to drastically change may be possible, but it’s certainly not an easy approach.
And most importantly, why force adaptation if you don’t need to? This is why our goal is to provide as close to natural sound input from the very beginning. We want to let recipients focus on fine-tuning their listening experience and improving their abilities for more challenging environments.
This can support rapid accommodation and adaption to hearing with a MED-EL CI. A number of studies have shown fast improvement in hearing ability with a MED-EL CI, with users often reaching very reasonable speech understanding or beyond within the first few months. Enabling recipients to experience their best benefit quickly is yet another advantage of providing a closer match to nature.
Natural Hearing: The Right Timing
Across the whole cochlea, you hear specific frequencies of sound based on where the cochlea is stimulated, which we have described above as place-pitch sound coding. This means you need to be in the right place for both the first and the second turn of the cochlea.
However, in the second turn of the cochlea, there is also a second type of sound coding for even more precision in natural hearing. In addition to place coding, the second turn of the cochlea uses time or rate coding. Basically, place-pitch coding looks at which hair cell is activated, while rate-pitch coding looks at how fast or slow that hair cell is switched on and off by a sound wave. This means you have to be at the right place and have the right timing.3,4,5,6,12
Understanding Natural Time Coding
This amazing natural time coding synchronizes the frequency of a sound wave to how often a nerve sends signals. This coding is called phase locking, because the timing of the auditory nerve signals (compound action potentials) is locked to the timing of the sound wave. For example, a tone with a frequency of 120 Hz will cause the hair cell at the 120 Hz location in the cochlea to trigger 120 auditory nerve signals per second. Your brain can then precisely understand this as a 120 Hz sound.
This rate coding is especially important for cochlear implant sound quality, because in the second turn, rate coding can override place coding. So even if you are at the right place, you still need to have the right timing.
For example, for a 120 Hz fundamental of male voice stimulated at the correct place in the cochlea, if you use a standard fixed stimulation rate of 800 electrical pulses per second, you will activate the nerve cells to send signals 800 times per second, and your brain would likely understand this as shifted upwards in pitch towards 800Hz. And this, of course, would have an unnatural effect on sound quality, is sometimes described as “pitch confusion”. Just like playing a vinyl record too fast, if you stimulate too fast, you make the sound unnaturally high-pitched.3,4,5,6
But if you slowed the stimulation down to 120 pulses per second to match the natural 120 Hz frequency, you stimulate the auditory nerve at the right rate and mimic the natural phase-locking, enabling a much more natural perception of sound.3,4,5,6
Why do we care about such precise details in the cochlea? With long MED-EL electrodes, you can reach the widest range of natural frequencies, up to the second turn where this rate coding is important to enable the closest to natural hearing.
For other companies that only offer short electrode arrays and only cover half of the cochlea (360°–450°), this rate coding doesn’t matter, because they can’t reach the second turn of the cochlea. And without the ability to provide accurate rate coding, it wouldn’t make sense for these other systems to have longer electrodes, because they still would likely not be able to experience accurate pitch perception in the lower frequencies.
If you can combine accurate place and also accurate timing information, you can provide a closer match to natural pitch perception. This is why we developed our unique rate-matched FineHearing technology.3,4,6,12,15
FineHearing: The Right Timing
FineHearing is the only sound coding for cochlear implants that instantly adapts the stimulation rate for electrode contacts in the second turn to match the natural frequencies of the incoming sounds. This means a 120 Hz sound is automatically coded with 120 stimulation pulses per second (pps), 144Hz at 144 pps, 275 Hz at 275 pps, and so on.
As mentioned earlier, FineHearing also creates up to 250 virtual stimulation channels for a richer, more natural sound perception across the whole array. And FineHearing even helps preserve interaural timing differences for better sound localization. This is a powerful combination that no other cochlear implant sound coding can match.12,19,24
By combining long electrode arrays for accurate place coding with FineHearing for accurate rate coding, you can achieve a more accurate, more natural stimulation of the whole cochlea. And by providing the closest to natural stimulation of the cochlea, you can enable the closest to natural hearing experience with a cochlear implant.1–20
Long MED-EL Electrode Arrays (Right Place) + FineHearing (Right Timing) = Enabling Closest to Natural Hearing
Closest to Natural Hearing
Better sound quality isn’t just a technical achievement—it’s a real benefit for everyday listening. Favorite sounds can sound more natural and familiar. Many of our recipients report being able to enjoy music, which would be difficult with a distorted sound quality.
More natural sound quality also means using our incredible natural auditory system as it was intended. This can make it easier for the brain to interpret complex listening environments, so your patients can hear better in noisy settings. 1–20
- Closest to natural sound quality
- Using the full potential of the natural cochlea
- Richer, fuller hearing experience
- More natural music quality/perception
- Better hearing in quiet and noise
- Improved sound localization
What Does a MED-EL Cochlear Implant Sound Like?
Much like learning to play a musical instrument, learning to hear with a cochlear implant takes time, practice, and patience. Every person’s hearing experience will be different. On activation day, many recipients may only hear beeps or unfamiliar sounds, while others may already be able to understand voices. Thankfully, this effort is worth it, because cochlear implants can effectively restore the ability to hear the sounds of life.
Many cochlear implant systems can provide reasonable speech understanding scores, especially in quiet settings. However, we at MED-EL do not believe that a cochlear implant should stop at “good enough”. Instead of only testing to see if recipients can regain functional speech understanding, we want to know that they are experiencing their best hearing in everyday life.
So how helpful is their MED-EL cochlear implant, and how close to natural is their hearing experience?
We asked more than 100 MED-EL CI users to rate how much they agree with the following statements, on a scale from 0/10 = “strongly disagree” to 10/10 = “strongly agree”. You can see their combined ratings below.29
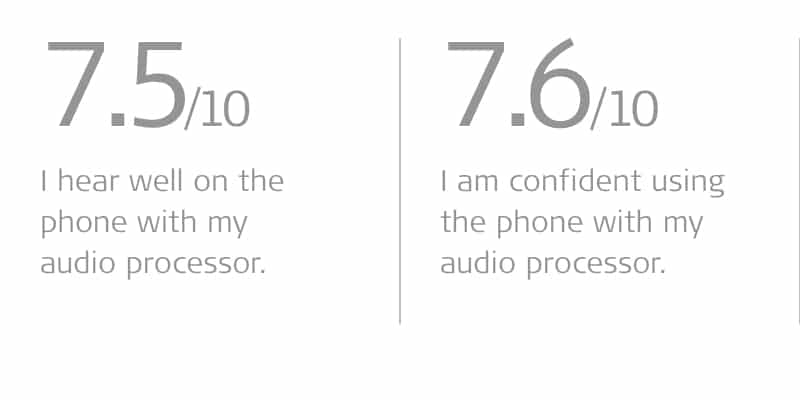
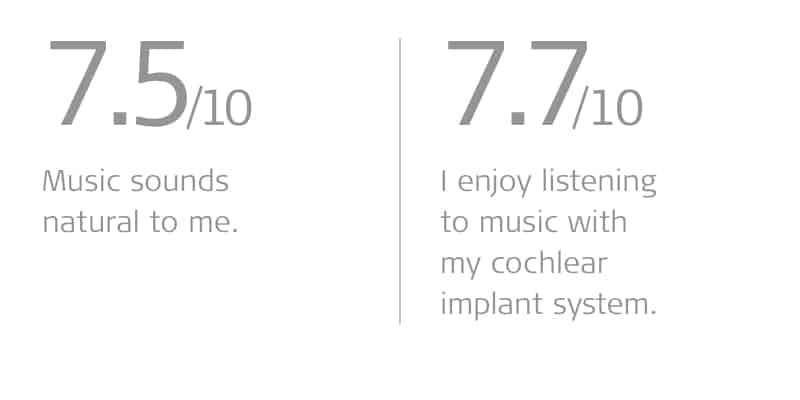
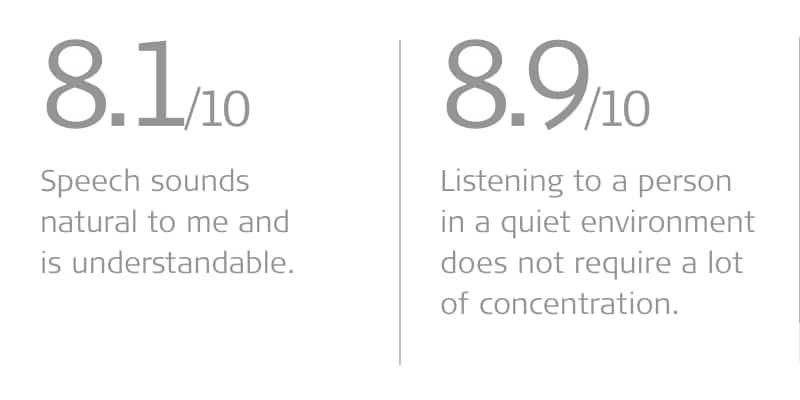
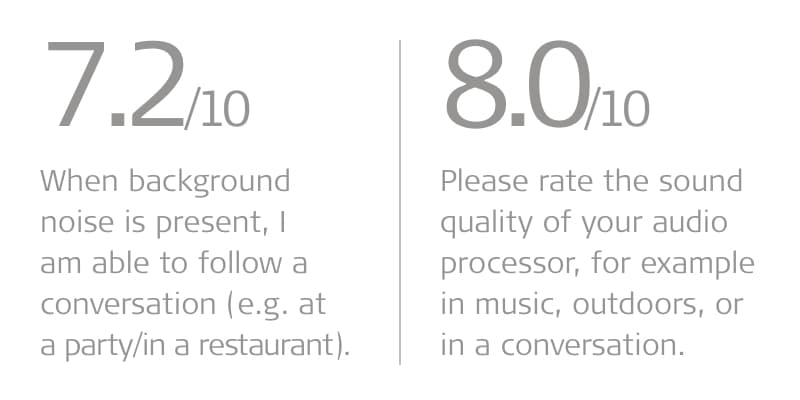
As you can see, cochlear implants can provide so much more than just being able to understand words. With MED-EL, cochlear implants can mean enjoying a lively conversation, picking up the phone with confidence, and rediscovering the joy of music.
Experiencing the more natural hearing can help restore the freedom to live independently, the confidence to face new challenges, and the happiness of truly reconnecting with friends and family.
Next Steps
So as we’ve seen, when it comes to sound quality, our unique closer to natural philosophy sets MED-EL apart from any other cochlear implant system.
But there are so many more reasons why our recipients love their MED-EL implant systems: proven reliability, Bluetooth connectivity, wireless charging, bimodal matching, all-in-one audio processors, and much more.
If you’d like to learn more about the advantages of MED-EL cochlear implants for your patients, let us know with our simple contact form. We’ll get you connected with your local MED-EL clinical support team to give you a hands-on tour of our systems and answer any questions you may have.
Don’t forget to subscribe to get regular updates from our MED-EL Professionals Blog!
References:
- Dorman, M.F., Cook Natale, S., Baxter, L., Zeitler, D.M., Carlson, M.L., Lorens, A., Skarzynski, H., Peters, J.P.M., Torres, J.H., & Noble, J.H. (2020). Approximations to the voice of a cochlear implant: explorations with single-sided deaf listeners. Trends Hear. 24:2331216520920079.
- Dorman, M. F., Natale, S. C., Butts, A. M., Zeitler, D. M. & Carlson, M. L. (2017). The Sound Quality of Cochlear Implants: Studies With Single-sided Deaf Patients. Otology & Neurotology, 38(8), e268–e273.
- Schatzer, R., Vermeire, K., Visser, D., Krenmayr, A., Kals, M., Voormolen, M., Van de Heyning, P., & Zierhofer, C. (2014). Electric-acoustic pitch comparisons in single-sided-deaf cochlear implant users: frequency-place functions and rate pitch. Hear Res., 309, 26–35.
- Rader, T., Döge, J., Adel, Y., Weissgerber, T., & Baumann, U. (2016). Place dependent stimulation rates improve pitch perception in cochlear implantees with single-sided deafness. Hear Res., 339, 94–103.
- Landsberger, D.M., Vermeire, K., Claes, A., Van Rompaey, V., & Van de Heyning, P. (2016). Qualities of single electrode stimulation as a function of rate and place of stimulation with a cochlear implant. Ear Hear., 37(3), 149–159.
- Prentiss, S., Staecker, H., & Wolford, B. (2014). Ipsilateral acoustic electric pitch matching: a case study of cochlear implantation in an up-sloping hearing loss with preserved hearing across multiple frequencies. Cochlear Implants Int., 15(3), 161–165.
- Landsberger, D.M., Svrakic, M., Roland, J.T. Jr., & Svirsky, M. (2015). The relationship between insertion angles, default frequency allocations, and spiral ganglion place pitch in cochlear implants. Ear Hear., 36(5), 207–213.
- Griessner, A., Schatzer, R., Steixner, V., Rajan, G. P., Zierhofer, C., & Távora-Vieira, D. (2021). Temporal Pitch Perception in Cochlear-Implant Users: Channel Independence in Apical Cochlear Regions. Trends in Hearing.
- Dorman, M. F., Natale, S. C., Zeitler, D. M., Baxter, L., & Noble, J. H. (2019). Looking for Mickey Mouse™ But Finding a Munchkin: The Perceptual Effects of Frequency Upshifts for Single-Sided Deaf, Cochlear Implant Patients. Journal of speech, language, and hearing research : JSLHR, 62(9), 3493–3499.
- Canfarotta, M. W., Dillon, M. T., Buss, E., Pillsbury, H. C., Brown, K. D., & O’Connell, B. P. (2020). Frequency-to-place mismatch: Characterizing variability and the influence on speech perception outcomes in cochlear implant recipients. Ear & Hearing, 41(5), 1349–1361.
- Canfarotta, M. W., Dillon, M. T., Buchman, C. A., Buss, E., O’Connell, B. P., Rooth, M. A., King, E. R., Pillsbury, H. C., Adunka, O. F., & Brown, K. D. (2020). Long‐term influence of electrode array length on speech recognition in cochlear implant users. The Laryngoscope, 131(4), 892–897.
- Roy, A.T., Carver, C., Jiradejvong, P., & Limb, C.J. (2015). Musical sound quality in cochlear implant users: A comparison in bass frequency perception between Fine Structure Processing and High-Definition Continuous Interleaved Sampling Strategies. Ear Hear., 36(5), 582–590.
- Li, H., Schart-Moren, N., Rohani, S., A., Ladak, H., M., Rask-Andersen, A., & Agrawal, S. (2020). Synchrotron Radiation-Based Reconstruction of the Human Spiral Ganglion: Implications for Cochlear Implantation. Ear Hear. 41(1).
- Buechner, A., Illg, A., Majdani, O., & Lenarz, T. (2017). Investigation of the effect of cochlear implant electrode length on speech comprehension in quiet and noise compared with the results with users of electro-acoustic-stimulation, a retrospective analysis. PLoS One. 12(5).
- Roy, A.T., Penninger, R.T., Pearl, M.S., Wuerfel, W., Jiradejvong, P., Carver, C., Buechner, A., & Limb, C.J. (2016). Deeper cochlear implant electrode insertion angle improves detection of musical sound quality deterioration related to bass frequency removal. Otol Neurotol., 37(2), 146–151.
- Dhanasingh, A. & Jolly, C. (2017). An overview of cochlear implant electrode array designs. Hear Res. 356: 93-103.
- Timm, M.E., Majdani, O., Weller, T., Windeler, M., Lenarz, T., Buechner, A. & Salcher, R.B. (2018). Patient specific selection of lateral wall cochlear implant electrodes based on anatomical indication ranges. PLoS ONE 13(10).
- McDermott, H., Sucher, C., & Simpson, A. (2009). Electro-acoustic stimulation. Acoustic and electric pitch comparisons. Audiol Neurootol., 14(1), 2–7.
- Harris, R.L., Gibson, W.P. Johnson, M., Brew, J., Bray, M., & Psarros, C. (2011). Intra-individual assessment of speech and music perception in cochlear implant users with contralateral Cochlear and MED-EL systems. Acta Otolaryngol., 131(12), 1270–1278.
- Analysis based on mean cochlear values and clinical data. Data on file.
- da Silva, J. C., Goffi-Gomez, M. V. S., Magalhães, A. T., Tsuji, R. K., & Bento, R. F. (2020). Is the spread of excitation width correlated to the speech recognition in cochlear implant users? European Archives of Oto-Rhino-Laryngology, 278(6), 1815–1820.
- Nobbe, A., Schleich, P., Zierhofer, C. & Nopp, P. (2007). Frequency discrimination with sequential or simultaneous stimulation in MED-EL cochlear implants. Acta Oto-Laryngologica, 127(12), 1266–1272.
- Buss, E., Dillon, M.T., Rooth, M.A., King, E.R., Deres, E.J., Buchman, C.A., Pillsbury, H.C., & Brown, K.D. (2018). Effect of cochlear implantation on binaural hearing in adults with unilateral hearing loss. Trends Hear. 22.
- Killan, C., Scally, A., Killan, E., Totten, C., & Raine, C. (2018). Factors affecting sound-source localization in children with simultaneous or sequential bilateral cochlear implants. Ear & Hearing, 40(4), 870–877.
- Vaerenberg, B., Govaerts, P.J., Stainsby, T., Nopp, P., Gualt, A., & Gnansia, D. (2014). A uniform graphical representation of intensity coding in current-generation cochlear implant systems. Ear Hear. 35(5): 533–543
- Pietsch, M., Schurzig, D., Salcher, R., Warnecke, A., Erfurt, P., Lenarz, T., &Kral, A. (2021) Variations in Microanatomy of the Human Modiolus: 2 Implications for Cochlear Implants.
- Saba, J. N., Ali, H. & Hansen, J. H. L. (2018). Formant priority channel selection for an “n-of-m” sound processing strategy for cochlear implants. The Journal of the Acoustical Society of America, 144(6), 3371–3380.
- Fuller, C. D., Gaudrain, E., Clarke, J. N., Galvin, J. J., Fu, Q. J., Free, R. H. & Başkent, D. (2014). Gender Categorization Is Abnormal in Cochlear Implant Users. Journal of the Association for Research in Otolaryngology, 15(6), 1037–1048.
- Survey of MED-EL cochlear implant recipients. 2019. Data on file.
MED-EL
Was this article helpful?
Thanks for your feedback.
Sign up for newsletter below for more.
Thanks for your feedback.
Please leave your message below.
CTA Form Success Message
Send us a message
Field is required
John Doe
Field is required
name@mail.com
Field is required
What do you think?
The content on this website is for general informational purposes only and should not be taken as medical advice. Please contact your doctor or hearing specialist to learn what type of hearing solution is suitable for your specific needs. Not all products, features, or indications shown are approved in all countries.
Cara Heers
November 05, 2024
I found this article interesting in general. Specifically, because I learned that rate coding contributes to a more natural sound.

MED-EL
Conversation
1 Comment