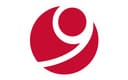
MED-EL
Published Mar 26, 2025
Can Reducing Tonotopic Mismatch Improve Hearing Outcomes for New CI Users?
There is growing evidence that cochlear implant recipients may benefit more from their devices if tonotopic mismatch is reduced. After providing an overview of recent research on tonotopic mismatch, this article explores how researchers and clinicians have been working to reduce tonotopic mismatch and improve hearing outcomes for MED-EL cochlear implant recipients.
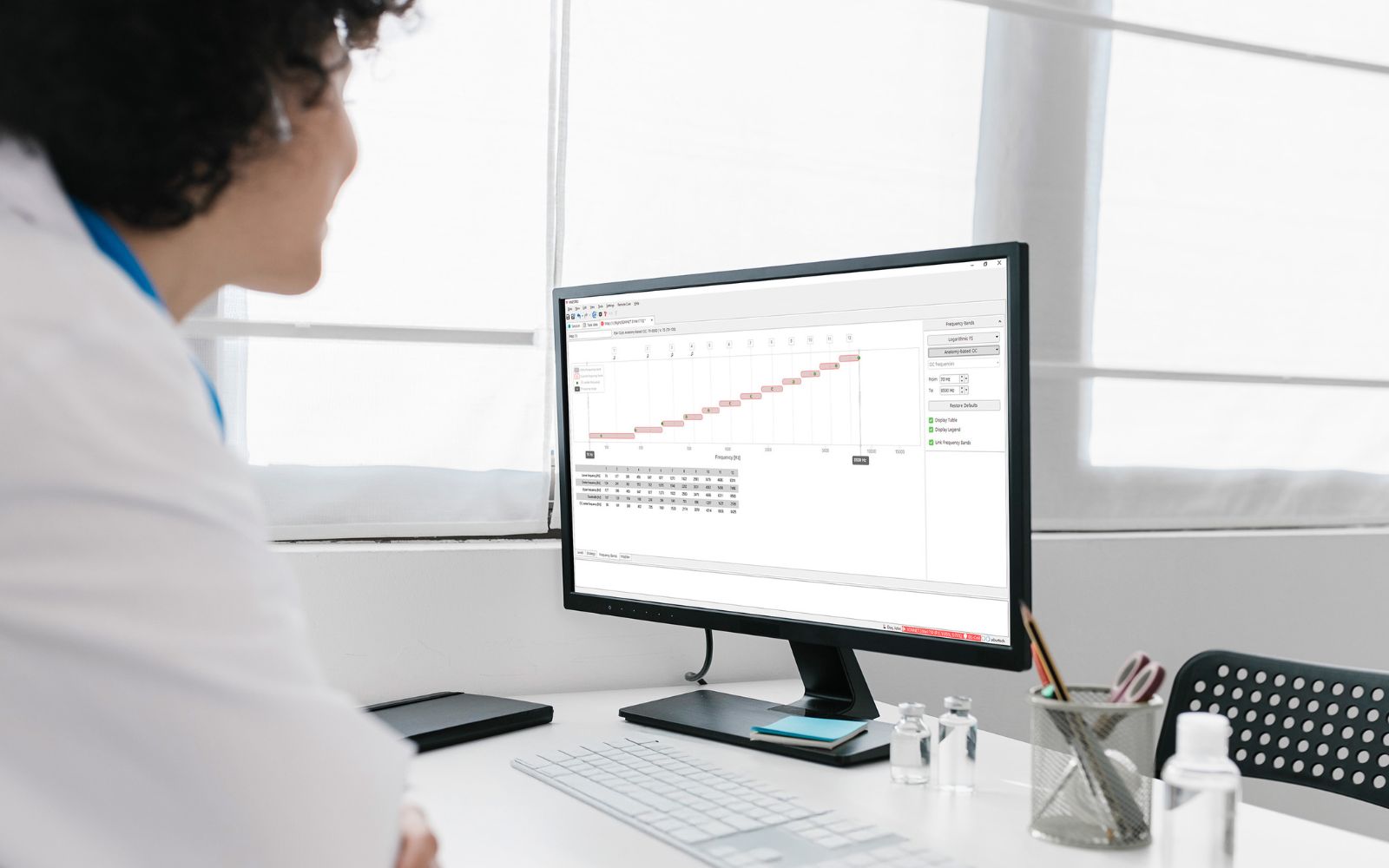
- 1 What Influences the Degree of Tonotopic Mismatch?
- 2 Default Frequency Filter Band Maps vs. Anatomy-Based Fitting
- 3 Anatomy-Based Fitting vs. Other Anatomical Place-Based Methods
- 4 Tonotopic Mismatch May Affect Different Populations of CI Users Differently
- 5 Research Findings: Tonotopic Mismatch & Hearing Outcomes in New CI Users
- 6 New Cochlear Implant Users Can Benefit From Anatomy-Based Fitting
- 7 Only MED-EL Provides Clinicians the Tools to Reduce Tonotopic Mismatch
Tonotopic mismatch has been gaining more attention among researchers and clinicians recently. Tonotopic mismatch, also known as place-pitch mismatch or frequency-to-place mismatch, is the discrepancy, within an implanted ear, between a cochlea’s natural frequency map and the frequency of an electrode contact assigned to that specific anatomic location with a cochlear implant.
It has been found that the degree of tonotopic mismatch varies significantly among cochlear implant and electric-acoustic stimulation (EAS) users,Canfarotta, M. W., Dillon, M. T., Buss, E., Pillsbury, H. C., Brown, K. D., & O’Connell, B. P. (2020). Frequency-to-Place Mismatch: Characterizing Variability and the Influence on Speech Perception Outcomes in Cochlear Implant Recipients. Ear and Hearing, 41(5), 1349–1361. https://doi.org/10.1097/aud.0000000000000864[5] and that tonotopic mismatch occurs with cochlear implants from all manufacturers Landsberger, D. M., Svrakic, M., Roland, J. T., & Svirsky, M. (2015). The Relationship Between Insertion Angles, Default Frequency Allocations, and Spiral Ganglion Place Pitch in Cochlear Implants. Ear and Hearing, 36(5), e207–e213. https://doi.org/10.1097/aud.0000000000000163[17] Venail, F., Mathiolon, C., Champfleur, S. M. de, Piron, J. P., Sicard, M., Villemus, F., Vessigaud, M. A., Sterkers-Artieres, F., Mondain, M., & Uziel, A. (2015). Effects of Electrode Array Length on Frequency-Place Mismatch and Speech Perception with Cochlear Implants. Audiology and Neurotology, 20(2), 102–111. https://doi.org/10.1159/000369333[29] and regardless of electrode type (perimodiolar or lateral wall).Mertens, G., Heyning, P. V. de, Vanderveken, O., Topsakal, V., & Rompaey, V. V. (2022). The smaller the frequency-to-place mismatch the better the hearing outcomes in cochlear implant recipients? European Archives of Oto-Rhino-Laryngology, 279(4), 1875–1883. https://doi.org/10.1007/s00405-021-06899-y[21] However, tonotopic mismatch is, in general, smaller with deeper electrode insertions and longer electrode arrays.
"Differences across devices were observed for the place of stimulation for frequencies below 650 Hz. Longer electrode arrays (i.e., the MED-EL STANDARD and FLEX28) demonstrated smaller deviations from the spiral ganglion map than the other electrode arrays. … A mismatch was observed between the predicted frequency and the default frequency provided by every electrode on all electrode arrays. The mismatch can be reduced by changing the default frequency allocations, inserting electrodes deeper into the cochlea, or allowing cochlear implant users to adapt to the mismatch."
Landsberger et al., 2015
Research shows that larger degrees of tonotopic mismatch may limit benefit for recipients when compared to peers with smaller degrees of mismatch, both in the first months during acclimatization and across the lifespan of cochlear implant usage.Canfarotta, M. W., Dillon, M. T., Buchman, C. A., Buss, E., O’Connell, B. P., Rooth, M. A., King, E. R., Pillsbury, H. C., Adunka, O. F., & Brown, K. D. (2021). Long‐Term Influence of Electrode Array Length on Speech Recognition in Cochlear Implant Users. The Laryngoscope, 131(4), 892–897. https://doi.org/10.1002/lary.28949[4]Canfarotta, M. W., Dillon, M. T., Buss, E., Pillsbury, H. C., Brown, K. D., & O’Connell, B. P. (2020). Frequency-to-Place Mismatch: Characterizing Variability and the Influence on Speech Perception Outcomes in Cochlear Implant Recipients. Ear and Hearing, 41(5), 1349–1361. https://doi.org/10.1097/aud.0000000000000864[5]Canfarotta, M. W., O’Connell, B. P., Buss, E., Pillsbury, H. C., Brown, K. D., & Dillon, M. T. (2020). Influence of Age at Cochlear Implantation and Frequency‐to‐Place Mismatch on Early Speech Recognition in Adults. Otolaryngology–Head and Neck Surgery, 162(6), 926–932. https://doi.org/10.1177/0194599820911707[6]Li, T., & Fu, Q.-J. (2010). Effects of spectral shifting on speech perception in noise. Hearing Research, 270(1–2), 81–88. https://doi.org/10.1016/j.heares.2010.09.005[20] Tonotopic mismatch has also been found to affect the performance of cochlear implant users particularly in complex listening environments, such as speech perception in noise. Lassaletta, L., Calvino, M., Sánchez-Cuadrado, I., & Gavilán, J. (2023). Does it make any sense to fit cochlear implants according to the anatomy-based fitting? Our experience with the first series of patients. Frontiers in Audiology and Otology, 1, 1298538. https://doi.org/10.3389/fauot.2023.1298538[19]Li, T., & Fu, Q.-J. (2010). Effects of spectral shifting on speech perception in noise. Hearing Research, 270(1–2), 81–88. https://doi.org/10.1016/j.heares.2010.09.005[20]
What Influences the Degree of Tonotopic Mismatch?
The degree of tonotopic mismatch depends on the relationship between anatomic, surgical, and CI-related factors. The factors that influence the degree of mismatch can be divided into four categories: (1) the unique anatomy of each ear, (2) the cochlear coverage of the CI electrode array, (3) surgery-related factors and electrode array type, and (4) the frequency filter adjustments made during cochlear implant fitting.
1. The Unique Anatomy of Each Ear
First, cochleae naturally vary significantly in size and shape. A systemic review of studies on cochlear anatomy found that cochlear duct lengths can range from 28.2–36.4 mm (n = 2252) and cochlear heights can range from 2.8–6.9 mm (n = 2098).Curtis, D. P., Baumann, A. N., & Jeyakumar, A. (2023). Variation in cochlear size: A systematic review. International Journal of Pediatric Otorhinolaryngology, 171, 111659. https://doi.org/10.1016/j.ijporl.2023.111659[8]
The natural variation in cochlear anatomy results in “significant variability in frequency-to-place mismatch among CI-alone and EAS users with default frequency filters, even between individuals implanted with the same array.”Canfarotta, M. W., Dillon, M. T., Buss, E., Pillsbury, H. C., Brown, K. D., & O’Connell, B. P. (2020). Frequency-to-Place Mismatch: Characterizing Variability and the Influence on Speech Perception Outcomes in Cochlear Implant Recipients. Ear and Hearing, 41(5), 1349–1361. https://doi.org/10.1097/aud.0000000000000864[5] Similarly, the uniqueness of each cochlea also leads to significant variation in angular insertion depths and cochlear coverage among users implanted with the same exact electrode array. Reiss, L. A. J., Turner, C. W., Karsten, S. A., & Gantz, B. J. (2014). Plasticity in human pitch perception induced by tonotopically mismatched electro-acoustic stimulation. Neuroscience, 256, 43–52. https://doi.org/10.1016/j.neuroscience.2013.10.024[24] Dutrieux, N., Quatre, R., Péan, V., & Schmerber, S. (2022). Correlation Between Cochlear Length, Insertion Angle, and Tonotopic Mismatch for MED-EL FLEX28 Electrode Arrays. Otology & Neurotology, 43(1), 48–55. https://doi.org/10.1097/mao.0000000000003337[12]
“Small cochlea size corresponded to higher insertion angle and reduction of tonotopic mismatch on a 28mm-long straight lateral wall electrode array. Tonotopic mismatch could be minimized preoperatively by choosing electrode arrays according to the individual cochlear morphology and postoperatively by appropriate frequency fitting.”
Dutrieux et al., 2022
“Selecting an electrode array length adapted to each individual’s cochlear anatomy may reduce frequency-place mismatch and thus improve speech perception.”
Venail et al., 2015
2. Cochlear Coverage of the Cochlear Implant Electrode
Second, cochlear coverage with the electrode array has been identified as one of the main factors that affects frequency-to-place mismatch.Aljazeeri, I., Hamed, N., Abdelsamad, Y., Sharif, T., Al‐Momani, M., & Hagr, A. (2022). Anatomy‐Based Frequency Allocation in Cochlear Implantation: The Importance of Cochlear Coverage. The Laryngoscope, 132(11), 2224–2231. https://doi.org/10.1002/lary.30004[3]Canfarotta, M. W., Dillon, M. T., Buss, E., Pillsbury, H. C., Brown, K. D., & O’Connell, B. P. (2020). Frequency-to-Place Mismatch: Characterizing Variability and the Influence on Speech Perception Outcomes in Cochlear Implant Recipients. Ear and Hearing, 41(5), 1349–1361. https://doi.org/10.1097/aud.0000000000000864[5] Hence, it is possible to reduce tonotopic mismatch preoperatively with lateral wall electrode arrays via electrode array selection. The key is selecting an electrode array that has the optimal length for each cochlea to achieve an adequate angular insertion depth and cochlear coverage.
Recent studies leveraging advanced high-resolution 3D synchrotron imaging have demonstrated that anatomical structures important for cochlear stimulation, such as the Rosenthal’s canal and spiral ganglion (SG), extend 1.5 to 2 turns in the cochlea.
The following graph depicts frequency distributions determined from synchrotron radiation phase-contrast imaging (SR-PCI) data. It shows the relationship between the anatomical place (in angular depth) and frequency (or pitch in Hertz) with respect to the organ of Corti (OC) and the spiral ganglion (SG). The results show that the angular depths associated with each tonotopic frequency are similar and overlap until roughly 600 degrees and 250 Hz, which is comparable to the average angular insertion depth of a FLEXSOFT electrode array. After that point, SG frequencies become more compressed in the cochlea’s apex, but the OC frequencies do not.
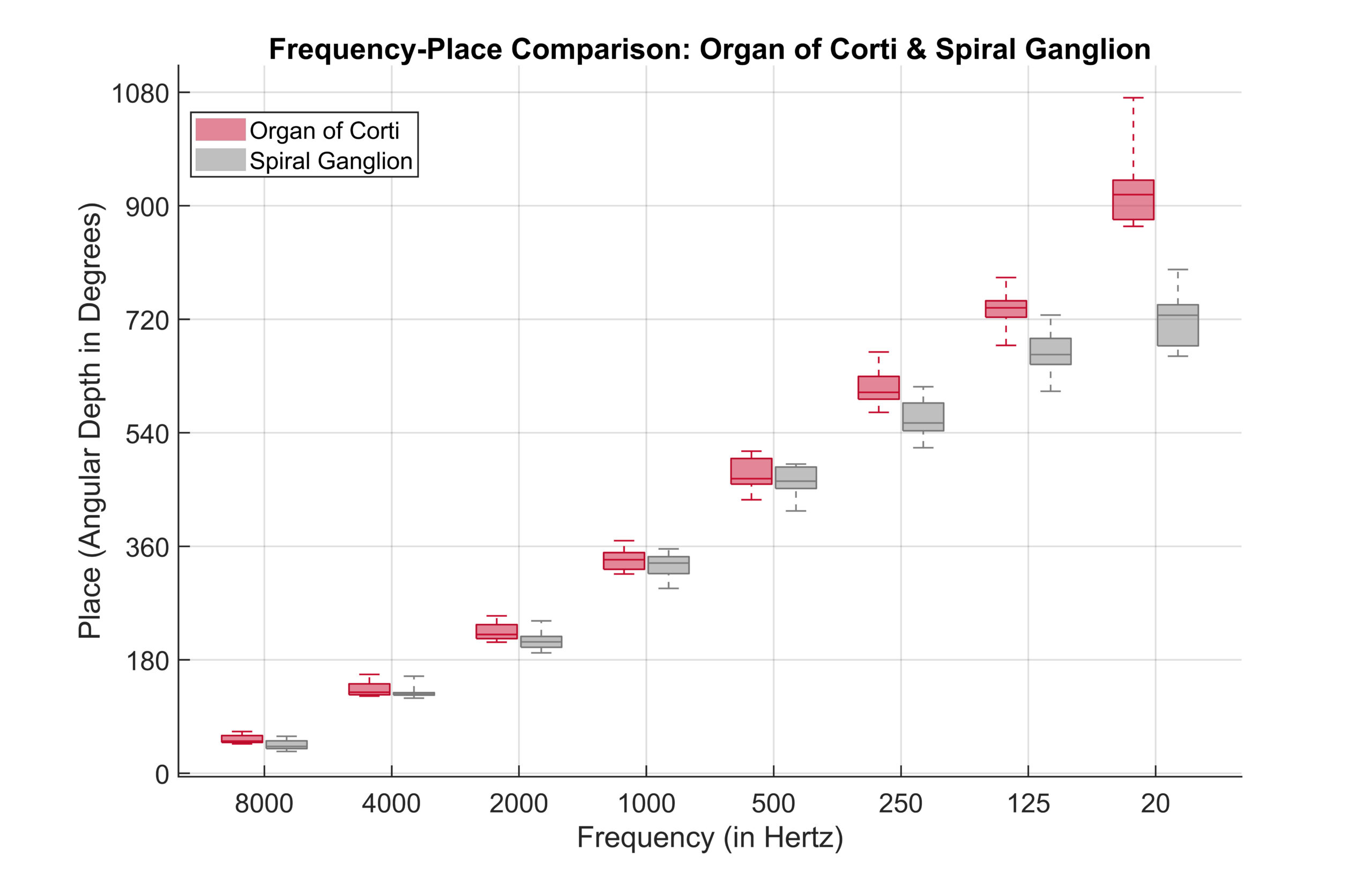
Adapted from Helpard et al., 2021.
“From a cochlear implant programming perspective, this means that the desired stimulation frequency in the basal and middle turns would be similar regardless of whether the cochlear implant stimulates the OC or SG.”
Helpard et al., 2021
Thus, in order to stimulate frequencies below 250 Hz at the natural corresponding tonotopic place, an electrode first must reach an angular depth beyond 540 degrees, or 1.5 turns. Then, when an electrode stimulates in the apical region beyond 1.5 turns, place-based coding can be used in combination with rate-based coding to provide additional frequency cues and information.
Only MED-EL Electrodes Can Stimulate Beyond 1.5 Turns
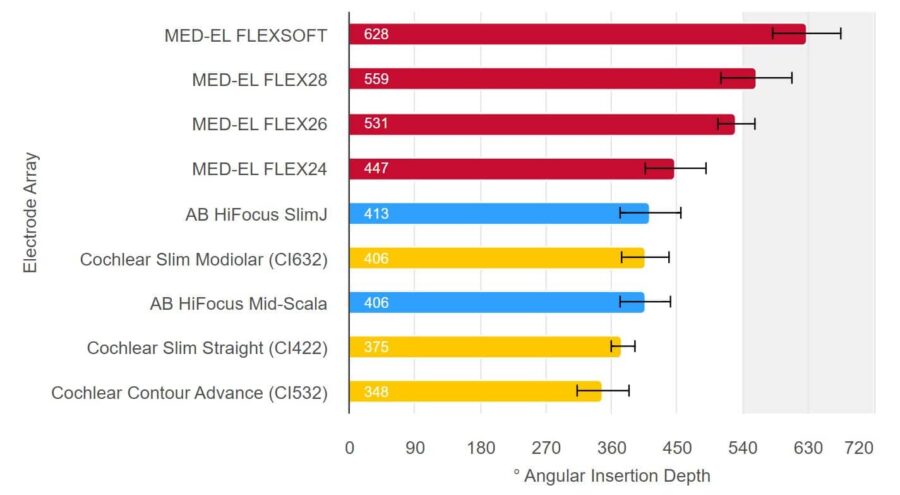
Source: Hassepass et al, 2014; Downing 2018; Ketterer et al. 2018; McJunkin et al. 2018; Skarzynski et al. 2018; Weller at al. 2023; Canforotta et al. 2021.6. Even the Smallest Cochlea Can Accommodate a FLEX Electrode
What Sets MED-EL's Electrodes Apart?
For more on how MED-EL CIs stimulate the apical region with place-based and rate-based coding, check out our newly published electrodes webpage.
Discover MoreResearchers examining tonotopic mismatch with respect to electrode length have observed the following:
"If the electrode array is too short, then tonotopic mismatch will be higher for frequency maps that cover at least the speech frequency range."
Canfarotta et al., 2020
"Frequency-to-place mismatch is typically small with a fully inserted long lateral wall array and default frequency filters; this is evident in the trend for less mismatch with longer arrays in the present study."
Canfarotta et al., 2020
"Aligning the frequency map of a CI to the tonotopic frequency map requires accurate measuring of both cochlear duct length and electrode contact locations. Both measurements are subject to significant variability between individuals.“
Mertens et al., 2022
3. Surgical Factors and Electrode Design
Third, surgery-related factors can also affect the degree of tonotopic mismatch. These include electrode array design type (perimodiolar, lateral wall, or mid-scala), position of the contacts relative to the stimulated neurons, and the surgical approach (round window vs. cochleostomy).Li, T., & Fu, Q.-J. (2010). Effects of spectral shifting on speech perception in noise. Hearing Research, 270(1–2), 81–88. https://doi.org/10.1016/j.heares.2010.09.005[20]
4. Adjustment of Frequency Filters During Fitting
Fourth, the frequency filters assigned to each electrode contact along electrode array (frequency map) during cochlear implant fitting can influence the degree of tonotopic mismatch. This, of course, is only relevant if the patient has an electrode long enough to stimulate the apical region. The next section explores what can be done to reduce tonotopic mismatch by adjusting the frequency filters to compensate for individual variation due to the other three factors mentioned assuming that the cochlear coverage is adequate.
Default Frequency Filter Band Maps vs. Anatomy-Based Fitting
The standard default frequency bands for each electrode contact are commonly assigned without the objective patient and ear-specific information that varies after every cochlear implantation is complete. Specifically, standard fitting methods do not take the factors that influence the degree of tonotopic mismatch mentioned above into account—natural anatomical variation, the implant’s electrode array length and angular insertion depth (cochlear coverage), and surgical factors. Each of those factors can influence the postoperative location of the electrode contacts relative to a patient’s cochlea, thus also influencing the resulting pitch perception from the cochlear implant.
Optimize Pitch Match With Real-World Data: No Guesswork With MED-EL CIs
The resulting sum of variation from the variable factors listed above is the postoperative location of the cochlear implant array’s electrode contacts with respect to individual ear’s cochlear anatomy. Until recently, computed tomography (CT) imaging, OTOPLAN, and MAESTRO’s anatomy-based fitting feature have been used to adjust for this in clinical practice, but now plain X-rays can also provide the postoperative information needed for anatomy-based fitting.Alahmadi, A., Abdelsamad, Y., Hafez, A., & Hagr, A. (2024). X-ray guided anatomy-based fitting: The validity of OTOPLAN. PLOS ONE, 19(11), e0313567. https://doi.org/10.1371/journal.pone.0313567[1]Alahmadi, A., Abdelsamad, Y., Thabet, E. M., Hafez, A., Alghamdi, F., Badr, K. M., Alghamdi, S., & Hagr, A. (2024). Advancing Cochlear Implant Programming: X-ray Guided Anatomy-Based Fitting. Otology & Neurotology, 45(2), 107–113. https://doi.org/10.1097/mao.0000000000004069[2]
“X-ray imaging provides a valid and easy-to-perform alternative to CT imaging, with less radiation exposure and lower costs. The radiographs showed excellent concordance with the CT-measured angular insertion depth and consequently with the central frequency for most electrode contacts.”
Alahmadi et al., 2024
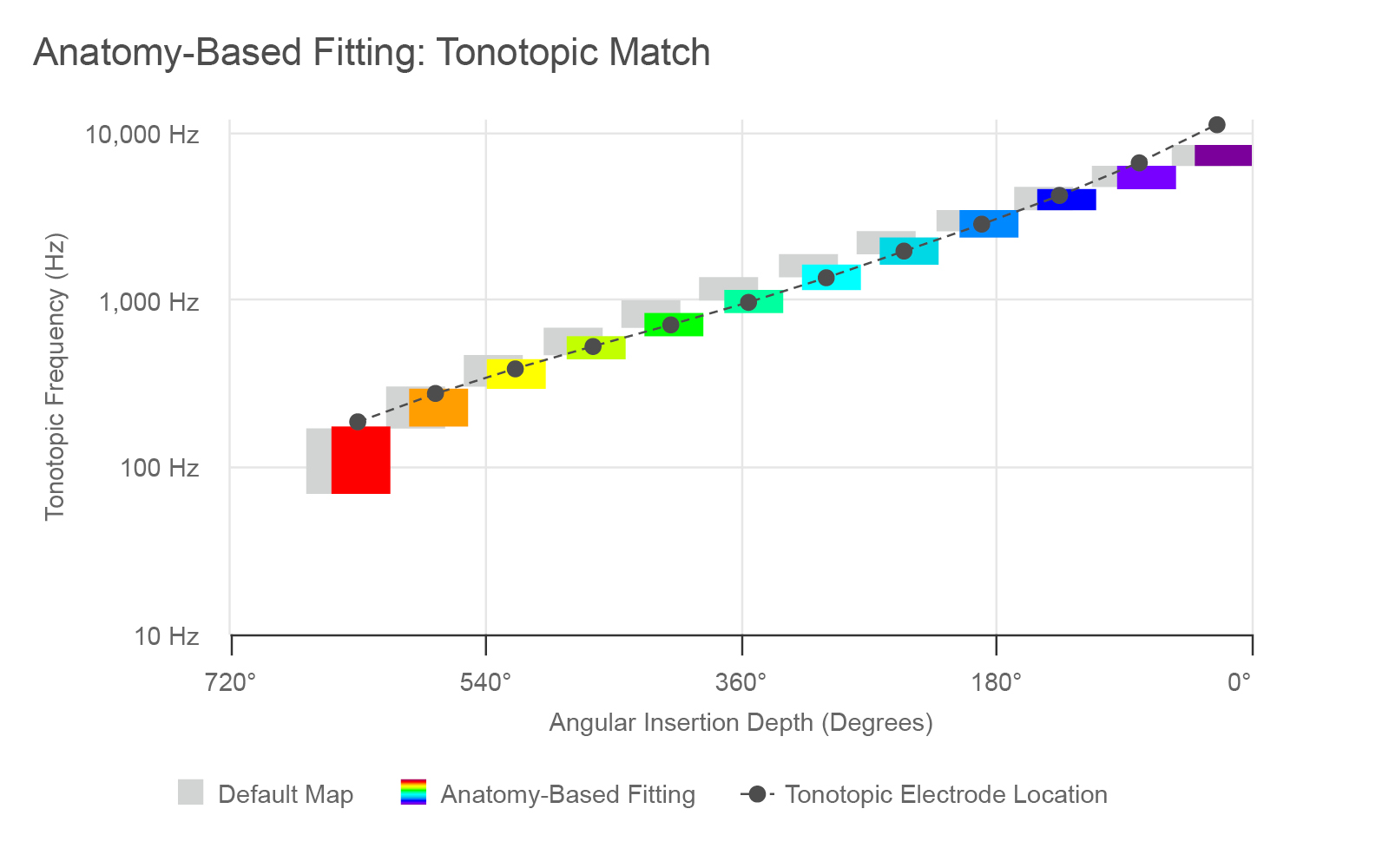
This graph above depicts an example of a MED-EL CI user with an insertion depth of 630 degrees, or 1.75 turns. The standard default frequency bands are shown with the gray bars. In contrast, a post-operative analysis using imaging data and OTOPLAN can be used to determine the angular insertion depth of the electrode array (the dark gray dotted line), the exact location of each electrode (the dots) and then calculate how these correspond to follow the natural tonotopic frequency map of each specific patient’s cochlea. Pitch, or the frequencies, are shown on the y-axis, and the place of stimulation is shown along the x-axis in degrees of angular insertion depth.
Thus, the tonotopic mismatch in the case shown above is the deviation between the light gray bars and dark gray dots. Once the anatomic location data from each ear is imported into MAESTRO (version 9 or later) and anatomy-based fitting is performed, the frequency bands can then be adjusted according to the exact locations of each electrode contact (the colored bars). In MAESTRO, the default map can be found under “Frequency Bands” and is labeled “Logarithmic FS”, along with the anatomy-based fitting option.
Anatomy-Based Fitting vs. Other Anatomical Place-Based Methods
Introduced in 2020 with MAESTRO 9.0, the anatomy-based fitting feature in MAESTRO has allowed clinicians and researchers to align the frequency filters based on Greenwood’s frequency-place map.Greenwood, D. D. (1990). A cochlear frequency-position function for several species—29 years later. The Journal of the Acoustical Society of America, 87(6), 2592–2605. https://doi.org/10.1121/1.399052[14] MAESTRO’s anatomy-based fitting algorithms focus on the critical speech frequency range from 950-3,000 Hz, adjusting the frequency filters based on each electrode’s anatomical position as well as the overall angular insertion depth determined by postoperative imaging.Dhanasingh, A., & Hochmair, I. (2021). Signal processing & audio processors. Acta Oto-Laryngologica, 141(S1), 106–134. https://doi.org/10.1080/00016489.2021.1888504[9]
It is important to point out that two different definitions exist for anatomy-based fitting in the field. While anatomy-based fitting is the name of the feature described above that is available in MED-EL’s MAESTRO software, anatomy-based fitting can sometimes be meant more broadly. ABF can be used to refer to other procedures that use postoperative anatomy information to derive filter band frequencies. In these cases, “anatomy-based fitting” and “place-based mapping” are among the most commonly used terms, but other terms may be used to describe the frequency mapping procedure.
In each of these cases, the frequency filters are adjusted using ear-specific anatomic imaging data that includes the postoperative location of the electrode array and its contacts. However, the frequency bands are not always derived using the algorithms of the anatomy-based fitting function (based on the Greenwood function) in MAESTRO. While many of the studies mentioned below used the MAESTRO software’s anatomy-based fitting function and algorithm to derive the frequency bands for mapping, not all studies have exactly used this approach. Some have used slightly different approaches but all have aimed to reduce tonotopic mismatch.
Tonotopic Mismatch May Affect Different Populations of CI Users Differently
Some researchers have hypothesized that tonotopic mismatch may especially impact postlingually-deafened cochlear implant recipients in the short-term following activation.Canfarotta, M. W., Dillon, M. T., Buss, E., Pillsbury, H. C., Brown, K. D., & O’Connell, B. P. (2020). Frequency-to-Place Mismatch: Characterizing Variability and the Influence on Speech Perception Outcomes in Cochlear Implant Recipients. Ear and Hearing, 41(5), 1349–1361. https://doi.org/10.1097/aud.0000000000000864[5]Thomas, M., Willis, S., Galvin, J. J., & Fu, Q.-J. (2022). Effects of tonotopic matching and spatial cues on segregation of competing speech in simulations of bilateral cochlear implants. PLoS ONE, 17(7), e0270759. https://doi.org/10.1371/journal.pone.0270759[28] Since they were used to normal acoustic hearing and the natural frequency-to-place match prior to experiencing hearing loss, they need time to adapt to the unfamiliar electric stimulation and place-pitch mismatch before they can fully benefit from their devices. Canfarotta, M. W., Dillon, M. T., Buss, E., Pillsbury, H. C., Brown, K. D., & O’Connell, B. P. (2020). Frequency-to-Place Mismatch: Characterizing Variability and the Influence on Speech Perception Outcomes in Cochlear Implant Recipients. Ear and Hearing, 41(5), 1349–1361. https://doi.org/10.1097/aud.0000000000000864[5]
Additionally, older cochlear implant recipients—who tend to perform worse in the first 6 months and may need more time to adjust to the new stimulation—could potentially benefit from reduced mismatch to optimize speech recognition outcomes in the months immediately following device activation.Canfarotta, M. W., O’Connell, B. P., Buss, E., Pillsbury, H. C., Brown, K. D., & Dillon, M. T. (2020). Influence of Age at Cochlear Implantation and Frequency‐to‐Place Mismatch on Early Speech Recognition in Adults. Otolaryngology–Head and Neck Surgery, 162(6), 926–932. https://doi.org/10.1177/0194599820911707[6] After the first months or year of cochlear implant use, many researchers believe that brain plasticity allows CI users to compensate for tonotopic mismatch after they become more familiar with stimulation from the CI.Peters, J. P. M., Bennink, E., & Zanten, G. A. van. (2019). Comparison of Place-versus-Pitch Mismatch between a Perimodiolar and Lateral Wall Cochlear Implant Electrode Array in Patients with Single-Sided Deafness and a Cochlear Implant. Audiology and Neurotology, 24(1), 38–48. https://doi.org/10.1159/000499154[22] However, research has also shown that in many CI users, this adaptation process can remain incomplete even after several years.Spiegel, J. L., Polterauer, D., Hempel, J.-M., Canis, M., Spiro, J. E., & Müller, J. (2022). Variation of the cochlear anatomy and cochlea duct length: analysis with a new tablet-based software. European Archives of Oto-Rhino-Laryngology, 279(4), 1851–1861. https://doi.org/10.1007/s00405-021-06889-0[25] Svirsky, M. A., Talavage, T. M., Sinha, S., Neuburger, H., & Azadpour, M. (2015). Gradual adaptation to auditory frequency mismatch. Hearing Research, 322, 163–170. https://doi.org/10.1016/j.heares.2014.10.008[26]
Therefore, the unique circumstances of each patient may affect the degree to which they may receive additional benefit from anatomy-based fitting. The listening modality also plays an important role. While place-pitch mismatch within an implanted ear has so far been the main focus of this article, interaural mismatch—or mismatch between both ears—can also affect hearing outcomes and binaural processing.Tan, C.-T., Martin, B., & Svirsky, M. A. (2017). Pitch Matching between Electrical Stimulation of a Cochlear Implant and Acoustic Stimuli Presented to a Contralateral Ear with Residual Hearing. Journal of the American Academy of Audiology, 28(03), 187–199. https://doi.org/10.3766/jaaa.15063[27] It is important to reduce mismatch and optimize electric hearing from the CI before matching with the contralateral ear—whether that ear has natural hearing, a hearing aid, or a second cochlear implant. More on matching electric stimulation from the CI to acoustic hearing on the contralateral side can be found in a section of our recent article, “Cochlear Implantation Outcomes in Adults With Unilateral Deafness: Speech Perception, Tinnitus, Sound Localization, and Quality of Life.”
Does Reducing Tonotopic Mismatch Affect Hearing Outcomes in New Cochlear Implant Users?
While a growing number of studies have focused on the relationship between tonotopic mismatch and hearing outcomes in experienced CI users, the remainder of this article will highlight the findings on hearing outcomes for fitting MED-EL cochlear implant users with tonotopic frequency maps vs. the default fitting across a variety of patient populations within the first 12 months after activation.
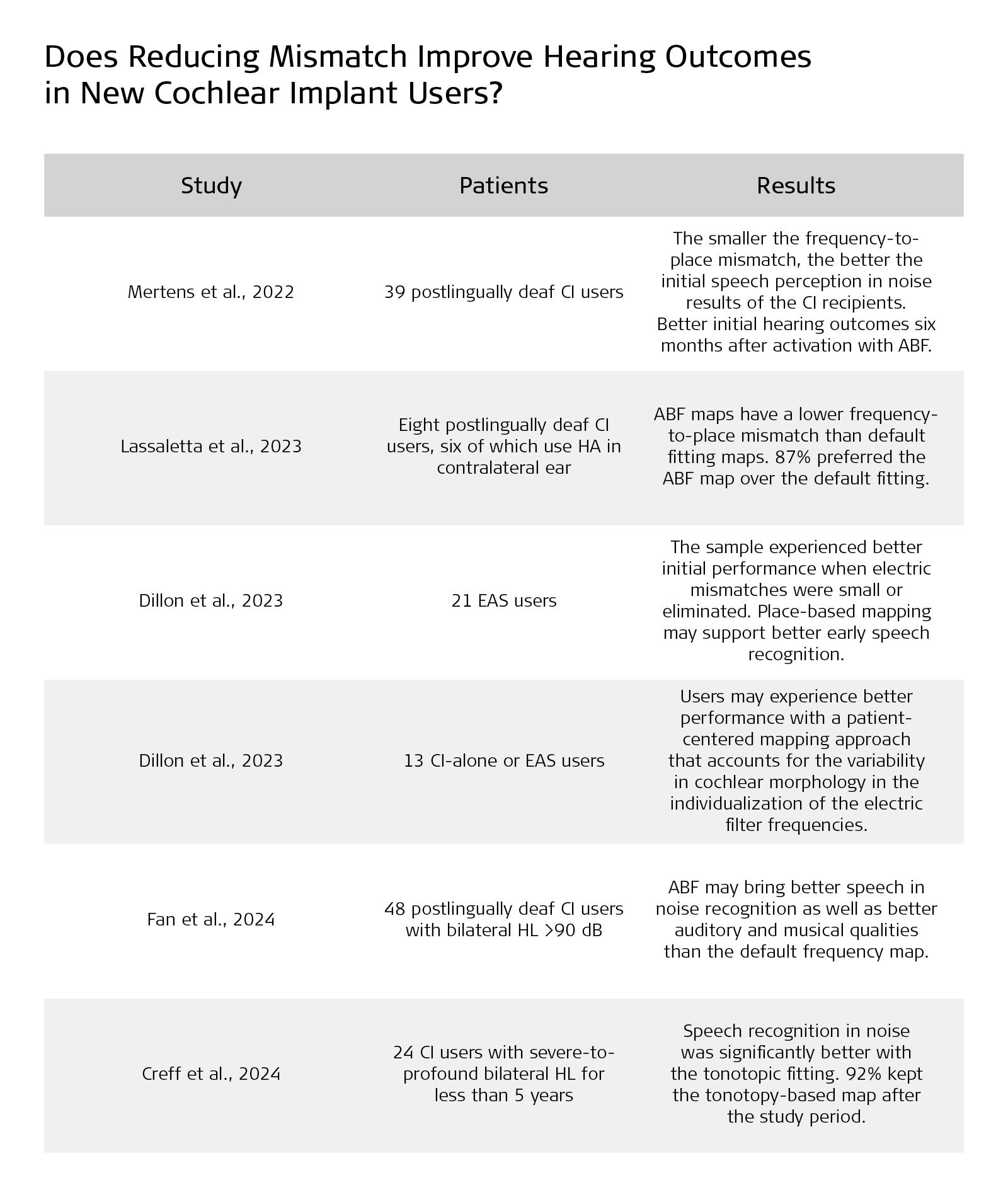
The Smaller the Frequency‑to‑Place Mismatch the Better the Hearing Outcomes in Cochlear Implant Recipients?
Mertens et al., 2022 investigated the effect of mismatch on speech perception in noise at six and 12 months after CI activation in 39 MED-EL recipients with FLEX28 electrodes.Mertens, G., Heyning, P. V. de, Vanderveken, O., Topsakal, V., & Rompaey, V. V. (2022). The smaller the frequency-to-place mismatch the better the hearing outcomes in cochlear implant recipients? European Archives of Oto-Rhino-Laryngology, 279(4), 1875–1883. https://doi.org/10.1007/s00405-021-06899-y[21] The study used patient CT imaging data and OTOPLAN software to apply anatomy-based fitting maps using the Greenwood function. Notably, the study also used the frequency mapping of the organ of Corti (OC), and it found a significant linear correlation between the mismatch and speech perception in noise after six months.
“The smaller the frequency-to-place mismatch, the better the initial hearing outcomes in CI recipients 6 months after activation.”
Mertens et al., 2022
However, the study also found that the significant effect of the mismatch disappeared after 12 months of CI use. Mertens et al. pointed out that, when the degree of mismatch is small, brain plasticity may be able to compensate for the mismatch, but that this does not mean that mismatch should not be reduced because large mismatches may limit performance and reduce sound quality. The study concluded that:
“To pursue better initial hearing outcomes and shorten the acclimatization process in CI recipients, the present results encourage further research to investigate the benefits of tonotopic fitting strategies. The software that was used in the present study is suitable to clinically estimate and use the tonotopic location of each electrode contact for tonotopic fitting.“
Mertens et al., 2022
Does It Make Any Sense to Fit Cochlear Implants According to Anatomy-Based Fitting? Our Experience With the First Series of Patients
A study by Lassaletta et al. provided additional evidence that new recipients may benefit from reduced tonotopic mismatch thanks to anatomy-based fitting maps created with postoperative data from OTOPLAN.Lassaletta, L., Calvino, M., Sánchez-Cuadrado, I., & Gavilán, J. (2023). Does it make any sense to fit cochlear implants according to the anatomy-based fitting? Our experience with the first series of patients. Frontiers in Audiology and Otology, 1, 1298538. https://doi.org/10.3389/fauot.2023.1298538[19] The study, focused on MED-EL SYNCHRONY ST recipients (n=8) with FLEX28 arrays (median angular insertion depth of 594◦), supported the findings from previous studies and found that no matter which fitting method is used deeper angular insertion depth of the most apical electrode is associated with smaller tonotopic mismatch in general. Results also indicated possible benefits from the anatomy-based fitting maps, which were found to lower mismatch by 20.7%.
“Within each subject ABF maps always had a lower mismatch than default fitting. ... ABF appears to work better with insertions between 540◦ (one and a half turns) and 740◦ (two turns) so that E1 is located between 340 and 85 Hz. With shallower insertions, lower frequencies may not be correctly stimulated despite adjusting the CI map to a tonotopic map.”
Lassaletta et al., 2023
At the end of the study, 87% of the recipients (all of them except one) preferred the ABF map over the default fitting. The authors reported that “the main reason is that ABF sounds less high-pitched than default fitting, which could be related to the observed mismatch reduction.” Despite finding similar hearing outcomes between the two fitting maps, they concluded that more data would be needed to verify the benefit of the ABF over default fitting in terms of speech and subjective hearing outcomes.
Influence of Electric Frequency-to-Place Mismatches on the Early Speech Recognition Outcomes for Electric-Acoustic Stimulation Users
Rather than looking into hearing outcomes with only electric stimulation from the CI, Dillon et al., 2023 explored the effect of reducing tonotopic mismatch on speech recognition outcomes in new users with both acoustic and electric stimulation in one ear.Dillon, M. T., Canfarotta, M. W., Buss, E., Rooth, M. A., Richter, M. E., Overton, A. B., Roth, N. E., Dillon, S. M., Raymond, J. H., Young, A., Pearson, A. C., Davis, A. G., Dedmon, M. M., Brown, K. D., & O’Connell, B. P. (2023). Influence of Electric Frequency-to-Place Mismatches on the Early Speech Recognition Outcomes for Electric–Acoustic Stimulation Users. American Journal of Audiology, 32(1), 251–260. https://doi.org/10.1044/2022_aja-21-00254[10]
The first publication to evaluate the potential benefit of using anatomical imaging data and OTOPLAN for fitting in this particular patient population, Dillon et al. assessed the influence of electric tonotopic mismatches on early speech recognition in EAS users. The study used a variant of anatomy-based fitting where the cochlear implant was fitted strictly tonotopically and each filter band was adjusted to the tonotopic frequency of the respective electrode regardless of the actual insertion depth of the electrodes. In addition, electrodes located in the acoustic hearing area were muted—steps that can be easily replicated using MAESTRO software. The place-based map group included six EAS users.
For subjects with default maps (n=15), electric mismatch at 1500 Hz ranged from 2 to 12.0 semitones. Poorer performance was observed for those with larger magnitudes of electric mismatch. This effect was observed through six months of EAS listening experience.
The authors concluded that their sample of EAS users experienced better speech recognition scores when electric mismatches were small or eliminated by using the place-based map. The data suggest the utility of methods that reduce electric mismatches, such as place-based mapping procedures. Further research is ongoing to determine whether these differences persist with long-term EAS use.
“Methods to minimize electric mismatches, such as place-based mapping procedures, may support better early speech recognition for [similar patient populations of EAS users].”
Dillon et al., 2023
Influence of the Frequency-to-Place Function on Recognition With Place-Based Cochlear Implant Maps
Dillon et al., 2023 compared speech recognition for MED-EL cochlear implant (CI) alone (n=10) and electric-acoustic stimulation users (n=3) 30 minutes after device activation.Dillon, M. T., Helpard, L., Brown, K. D., Selleck, A. M., Richter, M. E., Rooth, M. A., Thompson, N. J., Dedmon, M. M., Ladak, H. M., & Agrawal, S. (2023). Influence of the Frequency‐to‐Place Function on Recognition with Place‐Based Cochlear Implant Maps. The Laryngoscope, 133(12), 3540–3547. https://doi.org/10.1002/lary.30710[11] Aided speech recognition was evaluated listening with default maps or place-based maps using either a spiral ganglion (SG) or a new Synchrotron Radiation-Artificial Intelligence (SR‐AI) frequency-to-place method aligned to the to the organ of Corti’s tonotopicity. The order of map presentation was randomized across the participants. OTOPLAN and postoperative CT imaging were used to derive the place-based maps.
“On average, participants had better performance with the OC SR-AI place-based map as compared to the SG place-based map and the default map. A larger performance benefit was observed for EAS users than for CI-alone users. These pilot data suggest that EAS and CI-alone users may experience better performance with a patient-centered mapping approach that accounts for the variability in cochlear morphology (OC SR-AI frequency-to-place function) in the individualization of the electric filter frequencies (place-based mapping procedure).”
Dillon et al., 2023
Thus, the best outcomes were observed with maps that were individualized based on each ear’s anatomy, adjusted specifically to the tonotopic map using the organ of Corti frequencies.
Hearing Outcomes Following Cochlear Implantation With Anatomic or Default Frequency Mapping in Postlingual Deafness Adults
Fan et al. observed 48 new MED-EL CI users with FLEX28 or STANDARD electrodes (with angular insertion depths ranging from 469°–607°) who randomly received either an anatomy-based fitting map using OTOPLAN and MAESTRO, or a default fitting map when their devices were activated.Fan, X., Yang, T., Fan, Y., Song, W., Gu, W., Lu, X., Chen, Y., & Chen, X. (2024). Hearing outcomes following cochlear implantation with anatomic or default frequency mapping in postlingual deafness adults. European Archives of Oto-Rhino-Laryngology, 281(2), 719–729. https://doi.org/10.1007/s00405-023-08151-1[13] The subjects were all adults over the age of 18 with postlingual deafness with the amount of time between hearing loss and activation of their cochlear implants ranging from 6 to 50 years. After one year of cochlear implant use, both groups were tested on speech perception in quiet, speech perception in noise, sound field thresholds, the HISQUI19 questionnaire, and the APHAB questionnaire. The hearing tests were carried out in Mandarin Chinese with the recipients listening only through the ear with their cochlear implant.
Results showed that under noisy conditions, improvements in speech perception (SRS) were “significantly greater for anatomic group than the default group.” In quiet, however, there was no significant difference between the two groups in terms of mean hearing thresholds (0.5, 1, 2, and 4 kHz) and SRS. Modified questionnaires showed that auditory and musical quality were significantly better with anatomy-based fitting maps than those fitted with default maps.
“Compared with the default frequency procedure, the anatomic mapping frequency reallocation procedure ... [ABF] provided better hearing outcomes, especially SRS under noise conditions, and auditory and musical quality. These findings suggest that use of the anatomy-based fitting procedure may be optimal in patients unable to provide feedback, including small children.”
Fan et al., 2024
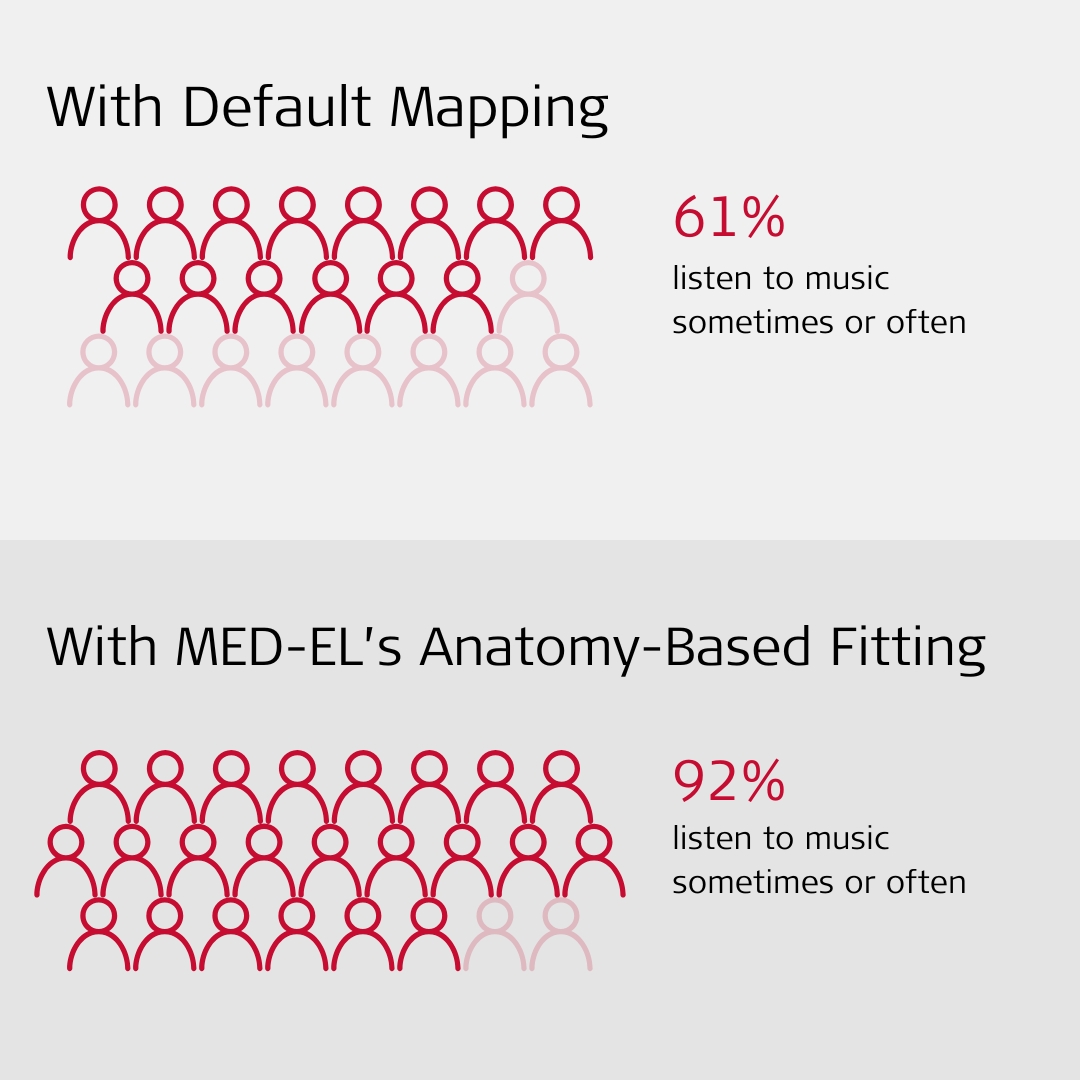
Notably, prior to cochlear implantation, 96% of the participants reported never listening to music since the majority were unable to hear or perceive the melodies and rhythm, and they found music to be uncomfortable and irritating. However, of those fitted with a MED-EL implant and the default frequency procedure, nine (39%) subjects reported never listening to music, and 14, or more than half of them (61%), said that they listen to music sometimes or often. In contrast, only two (8%) of the subjects reported never listening to music and 23 (92%) reported that they listen to music sometimes or often with the anatomy-based fitting procedure and their MED-EL CI.
Comparison of Tonotopic and Default Frequency Fitting for Speech Understanding in Noise in New Cochlear Implantees: A Prospective, Randomized, Double-Blind, Cross-Over Study
Creff et al.,2024 assessed the impact of a tonotopic map on speech perception in noise and quiet in new MED-EL cochlear implant users but for a shorter period after activation—the first six months.Creff, G., Lambert, C., Coudert, P., Pean, V., Laurent, S., & Godey, B. (2024). Comparison of Tonotopic and Default Frequency Fitting for Speech Understanding in Noise in New Cochlear Implantees: A Prospective, Randomized, Double-Blind, Cross-Over Study. Ear and Hearing, 45(1), 35–52. https://doi.org/10.1097/aud.0000000000001423[7] Implanted with either FLEX28 (n=16) or FLEXSOFT (n=10) electrode arrays with angular insertion depths ranging from 541°–708°, all of the subjects (n=26) assessed by Creff et al. were older than 18 years with bilateral severe-to-profound sensorineural hearing loss or had experienced complete hearing loss for less than 5 years. The mean angular insertion depth for the most apical electrode contact was 613 degrees.
CT scans and OTOPLAN were used to obtain and evaluate the postoperative anatomical data, and a mapping procedure was used based on the Greenwood function. Each participant was randomly assigned to receive a default map followed by a tonotopic map, or the other way around, and then speech tests were performed at regular intervals after six-week acclimation periods. The results of the Creff et al. study in 2024 confirm the findings that tonotopic fitting can improve speech recognition in noise.
“Speech recognition in noise was significantly better with the tonotopic fitting at all signal-to-noise ratio (SNR) levels tested. … Speech recognition in quiet (p = 0.66) and tonal audiometry (p = 0.203) did not significantly differ between the two settings. 92% of the participants kept the tonotopy-based map after the study period.”
Creff et al., 2024
Thus, compared with the default clinical frequency map, tonotopic frequency fitting improved hearing performance in noise without negatively affecting audiometric results in quiet.
New Cochlear Implant Users Can Benefit From Anatomy-Based Fitting
Preliminary research has found that within the first year of cochlear implant activation, cochlear implant users can benefit from place-based fitting methods, and many MED-EL cochlear implant recipients can benefit in the following ways from anatomy-based fitting:
- For new CI users who were post-lingually deafened, anatomy-based fitting can provide stimulation that is as close as possible to what they perceived before hearing loss, which reduces the time needed to adapt to their CI post-implantation. Creff, G., Lambert, C., Coudert, P., Pean, V., Laurent, S., & Godey, B. (2024). Comparison of Tonotopic and Default Frequency Fitting for Speech Understanding in Noise in New Cochlear Implantees: A Prospective, Randomized, Double-Blind, Cross-Over Study. Ear and Hearing, 45(1), 35–52. https://doi.org/10.1097/aud.0000000000001423[7]Fan, X., Yang, T., Fan, Y., Song, W., Gu, W., Lu, X., Chen, Y., & Chen, X. (2024). Hearing outcomes following cochlear implantation with anatomic or default frequency mapping in postlingual deafness adults. European Archives of Oto-Rhino-Laryngology, 281(2), 719–729. https://doi.org/10.1007/s00405-023-08151-1[13]Heitkötter, F. S., Krämer, B. A., Beule, A. G., & Rudack, C. (2024). Influence of Anatomy-Based Fitting in Cochlear Implant Users on Music Perception Using the Montreal Battery of Evaluation of Amusia. Otology & Neurotology. https://doi.org/10.1097/mao.0000000000004292[16]
- Anatomy-based tonotopic fitting maps provide speech perception benefits when compared to conventional default maps.Creff, G., Lambert, C., Coudert, P., Pean, V., Laurent, S., & Godey, B. (2024). Comparison of Tonotopic and Default Frequency Fitting for Speech Understanding in Noise in New Cochlear Implantees: A Prospective, Randomized, Double-Blind, Cross-Over Study. Ear and Hearing, 45(1), 35–52. https://doi.org/10.1097/aud.0000000000001423[7]Fan, X., Yang, T., Fan, Y., Song, W., Gu, W., Lu, X., Chen, Y., & Chen, X. (2024). Hearing outcomes following cochlear implantation with anatomic or default frequency mapping in postlingual deafness adults. European Archives of Oto-Rhino-Laryngology, 281(2), 719–729. https://doi.org/10.1007/s00405-023-08151-1[13]Walia, A., Shew, M. A., Varghese, J., Lefler, S. M., Bhat, A., Ortmann, A. J., Herzog, J. A., & Buchman, C. A. (2024). Electrocochleography-Based Tonotopic Map: II. Frequency-to-Place Mismatch Impacts Speech-Perception Outcomes in Cochlear Implant Recipients. Ear & Hearing. https://doi.org/10.1097/aud.0000000000001528[30]
- Anatomy-based fitting can improve sound quality and music perception for MED-EL CI users.Fan, X., Yang, T., Fan, Y., Song, W., Gu, W., Lu, X., Chen, Y., & Chen, X. (2024). Hearing outcomes following cochlear implantation with anatomic or default frequency mapping in postlingual deafness adults. European Archives of Oto-Rhino-Laryngology, 281(2), 719–729. https://doi.org/10.1007/s00405-023-08151-1[13]Helpard, L., Li, H., Rohani, S. A., Zhu, N., Rask-Andersen, H., Agrawal, S., & Ladak, H. M. (2021). An approach for individualized cochlear frequency mapping determined from 3D synchrotron radiation phase-contrast imaging. IEEE Transactions on Biomedical Engineering, 68(12), 3602-3611. https://doi.org/10.1109/tbme.2021.3080116[15]
- Most patients preferred listening with tonotopic maps.Creff, G., Lambert, C., Coudert, P., Pean, V., Laurent, S., & Godey, B. (2024). Comparison of Tonotopic and Default Frequency Fitting for Speech Understanding in Noise in New Cochlear Implantees: A Prospective, Randomized, Double-Blind, Cross-Over Study. Ear and Hearing, 45(1), 35–52. https://doi.org/10.1097/aud.0000000000001423[7]Heitkötter, F. S., Krämer, B. A., Beule, A. G., & Rudack, C. (2024). Influence of Anatomy-Based Fitting in Cochlear Implant Users on Music Perception Using the Montreal Battery of Evaluation of Amusia. Otology & Neurotology. https://doi.org/10.1097/mao.0000000000004292[16]Kurz, A., Herrmann, D., Müller-Graff, F.-T., Voelker, J., Hackenberg, S., & Rak, K. (2024). Anatomy-based fitting improves speech perception in noise for cochlear implant recipients with single-sided deafness. European Archives of Oto-Rhino-Laryngology, 1–13. https://doi.org/10.1007/s00405-024-08984-4[18]
- Most new cochlear implant users (79%), regardless of manufacturer, prefer tonotopic maps.Yang, A. W., Lee, K. F., Noller, M., Watson, N., Pillion, E. M., Riley, C. A., & Tolisano, A. M. (2022). Image-Guided Cochlear Implant Programming: A Systematic Review and Meta-analysis. Otology & Neurotology, 43(9), e924–e935. https://doi.org/10.1097/mao.0000000000003653[31]
- Anatomy-based fitting shows benefits when stimulation from the CI needs to be coordinated with acoustic hearing, either within the same ear (EAS users) or the normal acoustic hearing in the contralateral ear.
More studies investigating sound quality and music perception as well as others investigating clinical outcomes with experienced cochlear implant are underway.
Only MED-EL Provides Clinicians the Tools to Reduce Tonotopic Mismatch
Anatomy-based fitting is available in MAESTRO 9.0 or later.* For more about using OTOPLAN and X-ray or CT imaging data to provide your patients with anatomy-based fitting maps at your clinic, contact your local MED-EL representative.
Check out our recent article to learn how to boost clinical efficiency by incorporating Anatomy-Based Fitting and ESRT (Electrically Evoked Stapedial Reflex Threshold) into cochlear implant programming.
Subscribe to the MED-EL professionals blog to receive future updates on how to reduce mismatch for your patients.
For more in-depth information about research into place-based mapping and cochlear implant user hearing outcomes, you can watch our ExpertsONLINE on anatomy-based fitting below.
* Compatible with SONNET 2, SONNET 2 EAS, SONNET 3, SONNET 3 EAS and RONDO 3 audio processors.
References
-
[1]
Alahmadi, A., Abdelsamad, Y., Hafez, A., & Hagr, A. (2024). X-ray guided anatomy-based fitting: The validity of OTOPLAN. PLOS ONE, 19(11), e0313567. https://doi.org/10.1371/journal.pone.0313567
-
[2]
Alahmadi, A., Abdelsamad, Y., Thabet, E. M., Hafez, A., Alghamdi, F., Badr, K. M., Alghamdi, S., & Hagr, A. (2024). Advancing Cochlear Implant Programming: X-ray Guided Anatomy-Based Fitting. Otology & Neurotology, 45(2), 107–113. https://doi.org/10.1097/mao.0000000000004069
-
[3]
Aljazeeri, I., Hamed, N., Abdelsamad, Y., Sharif, T., Al‐Momani, M., & Hagr, A. (2022). Anatomy‐Based Frequency Allocation in Cochlear Implantation: The Importance of Cochlear Coverage. The Laryngoscope, 132(11), 2224–2231. https://doi.org/10.1002/lary.30004
-
[4]
Canfarotta, M. W., Dillon, M. T., Buchman, C. A., Buss, E., O’Connell, B. P., Rooth, M. A., King, E. R., Pillsbury, H. C., Adunka, O. F., & Brown, K. D. (2021). Long‐Term Influence of Electrode Array Length on Speech Recognition in Cochlear Implant Users. The Laryngoscope, 131(4), 892–897. https://doi.org/10.1002/lary.28949
-
[5]
Canfarotta, M. W., Dillon, M. T., Buss, E., Pillsbury, H. C., Brown, K. D., & O’Connell, B. P. (2020). Frequency-to-Place Mismatch: Characterizing Variability and the Influence on Speech Perception Outcomes in Cochlear Implant Recipients. Ear and Hearing, 41(5), 1349–1361. https://doi.org/10.1097/aud.0000000000000864
-
[6]
Canfarotta, M. W., O’Connell, B. P., Buss, E., Pillsbury, H. C., Brown, K. D., & Dillon, M. T. (2020). Influence of Age at Cochlear Implantation and Frequency‐to‐Place Mismatch on Early Speech Recognition in Adults. Otolaryngology–Head and Neck Surgery, 162(6), 926–932. https://doi.org/10.1177/0194599820911707
-
[7]
Creff, G., Lambert, C., Coudert, P., Pean, V., Laurent, S., & Godey, B. (2024). Comparison of Tonotopic and Default Frequency Fitting for Speech Understanding in Noise in New Cochlear Implantees: A Prospective, Randomized, Double-Blind, Cross-Over Study. Ear and Hearing, 45(1), 35–52. https://doi.org/10.1097/aud.0000000000001423
-
[8]
Curtis, D. P., Baumann, A. N., & Jeyakumar, A. (2023). Variation in cochlear size: A systematic review. International Journal of Pediatric Otorhinolaryngology, 171, 111659. https://doi.org/10.1016/j.ijporl.2023.111659
-
[9]
Dhanasingh, A., & Hochmair, I. (2021). Signal processing & audio processors. Acta Oto-Laryngologica, 141(S1), 106–134. https://doi.org/10.1080/00016489.2021.1888504
-
[10]
Dillon, M. T., Canfarotta, M. W., Buss, E., Rooth, M. A., Richter, M. E., Overton, A. B., Roth, N. E., Dillon, S. M., Raymond, J. H., Young, A., Pearson, A. C., Davis, A. G., Dedmon, M. M., Brown, K. D., & O’Connell, B. P. (2023). Influence of Electric Frequency-to-Place Mismatches on the Early Speech Recognition Outcomes for Electric–Acoustic Stimulation Users. American Journal of Audiology, 32(1), 251–260. https://doi.org/10.1044/2022_aja-21-00254
-
[11]
Dillon, M. T., Helpard, L., Brown, K. D., Selleck, A. M., Richter, M. E., Rooth, M. A., Thompson, N. J., Dedmon, M. M., Ladak, H. M., & Agrawal, S. (2023). Influence of the Frequency‐to‐Place Function on Recognition with Place‐Based Cochlear Implant Maps. The Laryngoscope, 133(12), 3540–3547. https://doi.org/10.1002/lary.30710
-
[12]
Dutrieux, N., Quatre, R., Péan, V., & Schmerber, S. (2022). Correlation Between Cochlear Length, Insertion Angle, and Tonotopic Mismatch for MED-EL FLEX28 Electrode Arrays. Otology & Neurotology, 43(1), 48–55. https://doi.org/10.1097/mao.0000000000003337
-
[13]
Fan, X., Yang, T., Fan, Y., Song, W., Gu, W., Lu, X., Chen, Y., & Chen, X. (2024). Hearing outcomes following cochlear implantation with anatomic or default frequency mapping in postlingual deafness adults. European Archives of Oto-Rhino-Laryngology, 281(2), 719–729. https://doi.org/10.1007/s00405-023-08151-1
-
[14]
Greenwood, D. D. (1990). A cochlear frequency-position function for several species—29 years later. The Journal of the Acoustical Society of America, 87(6), 2592–2605. https://doi.org/10.1121/1.399052
-
[15]
Helpard, L., Li, H., Rohani, S. A., Zhu, N., Rask-Andersen, H., Agrawal, S., & Ladak, H. M. (2021). An approach for individualized cochlear frequency mapping determined from 3D synchrotron radiation phase-contrast imaging. IEEE Transactions on Biomedical Engineering, 68(12), 3602-3611. https://doi.org/10.1109/tbme.2021.3080116
-
[16]
Heitkötter, F. S., Krämer, B. A., Beule, A. G., & Rudack, C. (2024). Influence of Anatomy-Based Fitting in Cochlear Implant Users on Music Perception Using the Montreal Battery of Evaluation of Amusia. Otology & Neurotology. https://doi.org/10.1097/mao.0000000000004292
-
[17]
Landsberger, D. M., Svrakic, M., Roland, J. T., & Svirsky, M. (2015). The Relationship Between Insertion Angles, Default Frequency Allocations, and Spiral Ganglion Place Pitch in Cochlear Implants. Ear and Hearing, 36(5), e207–e213. https://doi.org/10.1097/aud.0000000000000163
-
[18]
Kurz, A., Herrmann, D., Müller-Graff, F.-T., Voelker, J., Hackenberg, S., & Rak, K. (2024). Anatomy-based fitting improves speech perception in noise for cochlear implant recipients with single-sided deafness. European Archives of Oto-Rhino-Laryngology, 1–13. https://doi.org/10.1007/s00405-024-08984-4
-
[19]
Lassaletta, L., Calvino, M., Sánchez-Cuadrado, I., & Gavilán, J. (2023). Does it make any sense to fit cochlear implants according to the anatomy-based fitting? Our experience with the first series of patients. Frontiers in Audiology and Otology, 1, 1298538. https://doi.org/10.3389/fauot.2023.1298538
-
[20]
Li, T., & Fu, Q.-J. (2010). Effects of spectral shifting on speech perception in noise. Hearing Research, 270(1–2), 81–88. https://doi.org/10.1016/j.heares.2010.09.005
-
[21]
Mertens, G., Heyning, P. V. de, Vanderveken, O., Topsakal, V., & Rompaey, V. V. (2022). The smaller the frequency-to-place mismatch the better the hearing outcomes in cochlear implant recipients? European Archives of Oto-Rhino-Laryngology, 279(4), 1875–1883. https://doi.org/10.1007/s00405-021-06899-y
-
[22]
Peters, J. P. M., Bennink, E., & Zanten, G. A. van. (2019). Comparison of Place-versus-Pitch Mismatch between a Perimodiolar and Lateral Wall Cochlear Implant Electrode Array in Patients with Single-Sided Deafness and a Cochlear Implant. Audiology and Neurotology, 24(1), 38–48. https://doi.org/10.1159/000499154
-
[23]
Reiss, L. A. J., Turner, C. W., Erenberg, S. R., & Gantz, B. J. (2007). Changes in Pitch with a Cochlear Implant Over Time. Journal for the Association for Research in Otolaryngology, 8(2), 241–257. https://doi.org/10.1007/s10162-007-0077-8
-
[24]
Reiss, L. A. J., Turner, C. W., Karsten, S. A., & Gantz, B. J. (2014). Plasticity in human pitch perception induced by tonotopically mismatched electro-acoustic stimulation. Neuroscience, 256, 43–52. https://doi.org/10.1016/j.neuroscience.2013.10.024
-
[25]
Spiegel, J. L., Polterauer, D., Hempel, J.-M., Canis, M., Spiro, J. E., & Müller, J. (2022). Variation of the cochlear anatomy and cochlea duct length: analysis with a new tablet-based software. European Archives of Oto-Rhino-Laryngology, 279(4), 1851–1861. https://doi.org/10.1007/s00405-021-06889-0
-
[26]
Svirsky, M. A., Talavage, T. M., Sinha, S., Neuburger, H., & Azadpour, M. (2015). Gradual adaptation to auditory frequency mismatch. Hearing Research, 322, 163–170. https://doi.org/10.1016/j.heares.2014.10.008
-
[27]
Tan, C.-T., Martin, B., & Svirsky, M. A. (2017). Pitch Matching between Electrical Stimulation of a Cochlear Implant and Acoustic Stimuli Presented to a Contralateral Ear with Residual Hearing. Journal of the American Academy of Audiology, 28(03), 187–199. https://doi.org/10.3766/jaaa.15063
-
[28]
Thomas, M., Willis, S., Galvin, J. J., & Fu, Q.-J. (2022). Effects of tonotopic matching and spatial cues on segregation of competing speech in simulations of bilateral cochlear implants. PLoS ONE, 17(7), e0270759. https://doi.org/10.1371/journal.pone.0270759
-
[29]
Venail, F., Mathiolon, C., Champfleur, S. M. de, Piron, J. P., Sicard, M., Villemus, F., Vessigaud, M. A., Sterkers-Artieres, F., Mondain, M., & Uziel, A. (2015). Effects of Electrode Array Length on Frequency-Place Mismatch and Speech Perception with Cochlear Implants. Audiology and Neurotology, 20(2), 102–111. https://doi.org/10.1159/000369333
-
[30]
Walia, A., Shew, M. A., Varghese, J., Lefler, S. M., Bhat, A., Ortmann, A. J., Herzog, J. A., & Buchman, C. A. (2024). Electrocochleography-Based Tonotopic Map: II. Frequency-to-Place Mismatch Impacts Speech-Perception Outcomes in Cochlear Implant Recipients. Ear & Hearing. https://doi.org/10.1097/aud.0000000000001528
-
[31]
Yang, A. W., Lee, K. F., Noller, M., Watson, N., Pillion, E. M., Riley, C. A., & Tolisano, A. M. (2022). Image-Guided Cochlear Implant Programming: A Systematic Review and Meta-analysis. Otology & Neurotology, 43(9), e924–e935. https://doi.org/10.1097/mao.0000000000003653
References
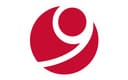
MED-EL
Was this article helpful?
Thanks for your feedback.
Sign up for newsletter below for more.
Thanks for your feedback.
Please leave your message below.
CTA Form Success Message
Send us a message
Field is required
John Doe
Field is required
name@mail.com
Field is required
What do you think?
The content on this website is for general informational purposes only and should not be taken as medical advice. Please contact your doctor or hearing specialist to learn what type of hearing solution is suitable for your specific needs. Not all products, features, or indications shown are approved in all countries.
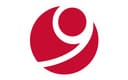
MED-EL
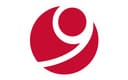
MED-EL